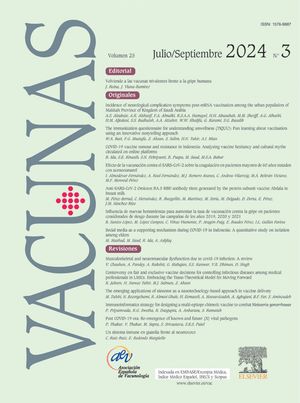
Editado por: Dra. Núria Torner CIBER Epidemiologia y Salud Publica CIBERESP Unitat de Medicina Preventiva i Salut Pública Departament de Medicina, Universitat de Barcelona
Más datosThe emergence of the novel coronavirus-based illness, COVID-19, in late 2019 marked a watershed moment in modern history. Initially identified in Wuhan, China, this viral epidemic swiftly transformed into a global pandemic, affecting worldwide. The World Health Organization (WHO) officially labeled the virus as “Severe Acute Respiratory Syndrome Coronavirus 2” (SARS-CoV-2) and the ensuing disease as Coronavirus Disease 2019 (COVID-19) on February 11, 2020. The spectrum of effects induced by COVID-19 encompasses a diverse array of conditions, ranging from sensory disturbances like anosmia and ageusia to more intricate manifestations, including headaches and, alarmingly, multiorgan failure and neuromuscular dysfunction, and even deaths were reported. COVID-19 triggers a vigorous inflammatory response characterized by the overproduction of pro-inflammatory cytokines. “Cytokine storm” significantly underwrites the worsening of the condition of patients, resulting in abnormalities such as ARDS. The major reason behind mortality from COVID-19 is respiratory failure instigated by ARDS. Throughout the early respiratory contamination, SARS-CoV-2 was hypothesized to target type-II pneumocytes lining the respiratory passage that specifically expressed TMPRSS2 and ACE2 receptors. The extra-pulmonary consequences of COVID-19 have been linked to the occurrence of these receptors in the skeletomuscular and brain tissues. As a result, it is condemning to comprehend the unique pathophysiological mechanisms behind the virus's manner of invasion in the tissues and also the disease's consequences. In this article, we focus on the complications caused by COVID-19 on musculoskeletal and neural tissues, the route of invasion, and the future perspective of dealing with the receptors whose expression leads to COVID-19 infection.
La aparición de la nueva enfermedad basada en el coronavirus, COVID-19, a finales de 2019 marcó un momento decisivo en la historia moderna. Inicialmente identificada en Wuhan, China, esta epidemia viral se transformó rápidamente en una pandemia global que afectó a todo el mundo. La Organización Mundial de la Salud (OMS) etiquetó oficialmente el virus como “Coronavirus 2 del síndrome respiratorio agudo severo” (SARS-CoV-2) y la enfermedad resultante como Enfermedad por coronavirus 2019 (COVID-19) el 11 de febrero de 2020. El espectro de efectos La enfermedad inducida por la COVID-19 abarca una amplia gama de afecciones, que van desde alteraciones sensoriales como la anosmia y la ageusia hasta manifestaciones más complejas, como dolores de cabeza y, de manera alarmante, insuficiencia multiorgánica y disfunción neuromuscular, e incluso se notificaron muertes. COVID-19 desencadena una vigorosa respuesta inflamatoria caracterizada por la sobreproducción de citocinas proinflamatorias. La “tormenta de citocinas” contribuye significativamente al empeoramiento de la condición de los pacientes, lo que resulta en anomalías como el SDRA. La principal razón detrás de la mortalidad por COVID-19 es la insuficiencia respiratoria provocada por el SDRA. Durante la infección respiratoria temprana, se planteó la hipótesis de que el SARS-CoV-2 se dirigía a los neumocitos tipo II que recubrían las vías respiratorias y que expresaban específicamente los receptores TMPRSS2 y ACE2. Las consecuencias extrapulmonares del COVID-19 se han relacionado con la aparición de estos receptores en los tejidos esqueletomusculares y cerebrales. Por lo tanto, es difícil comprender los mecanismos fisiopatológicos únicos detrás de la forma en que el virus invade los tejidos y también las consecuencias de la enfermedad. En este artículo nos centramos en las complicaciones que provoca la COVID-19 en los tejidos musculoesqueléticos y neurales, la ruta de invasión y la perspectiva futura de abordar los receptores cuya expresión conduce a la infección por COVID-19.
The Coronavirus 2019 (COVID-19) triggered Severe Acute Respiratory Syndrome Coronavirus 2 (SARS-CoV-2), which is contagious and was primarily recognized in Wuhan, China. In no time, it covered the entire area of the world and led to a pandemic situation.1,2 According to a WHO report, more than 526 million cases have been documented globally to date. International research teams are working to find out the mechanism of infection. Although many of the queries have been answered, there are still many unanswered. The first human coronavirus was discovered in 1965, which mostly showed symptoms of the common cold in infected people. COVID-19 has an average incubation period of 5 days.3 But many cases have been reported where the patients remained asymptomatic. The disease is caused when a person inhales air contaminated with droplets containing coronavirus. Through genetic analysis, it has been learned that the coronavirus infecting humans shows almost 96% parallelism with the bat coronavirus (BatCoV RaTG13).4 The coronavirus genome is single positive-stranded RNA and 4 structural proteins, namely, nucleocapsid protein (N), envelope protein (E), membrane glycoprotein (M), and spike protein (S), and are present. As reported in December 2021, there are 5 main variants of SARS-CoV-2, which are listed as Alpha (UK variant), Beta (South African variant), Delta (Indian variant), Gamma (Brazil variant), and Omicron.5 COVID-19 severity may be loosely categorized into 3 classes on the foundation of the acuteness of the primary infection. Mild symptoms of COVID-19, which account for most of the cases, almost 80%, are distinguished by indications like fatigue, cough, fever, gastrointestinal trouble, malaise, headaches, ageusia, anosmia, dyspnoea, and hypoxia. Patients with minor COVID-19 symptoms may or may not look for therapeutic help and may or may not present with mild pneumonia. Because of respiratory difficulties, severely ill patients must be hospitalized for infection treatment.6–9 Therefore, several antimicrobial drugs were used in hospitals on an empirical basis to prevent infection. Patients (approx. 6%) have shown critical symptoms like cardiac arrest, respiratory failure, and multiorgan dysfunction. Critically ill patients with respiratory failure require mechanical ventilator assistance.10
SARS-CoV-1 and CoV-2 have a significant percentage of parallelism in their genomic sequences.11,12 Both enter cells by attaching with ACE2 (angiotensin-converting enzyme 2), which is mediated through TMPRSS2 (transmembrane protease, serine 2).13 After attachment with the receptor, TMPRSS2 proteolytically cleaves the S protein of the virus, releasing a leader sequence that directs viral and human cell membrane fusion, and as a result, the viral RNA gets transferred into the cytosol of the human cell. This leads to viral RNA replication and viral protein translation, which results in the formation of virions. These potential infection-causing cells are then released by infected cells through exocytosis.14 This makes it possible for additional viruses to be produced, significantly impairs essential cellular processes, and eventually triggers apoptosis. Human proteins and processes involved in ubiquitin ligases, intracellular vesicle trafficking, nuclear transport, inflammatory signaling, mitochondrial respiration, and cytoskeletal integrity are among those probably to be aimed at by SARS-CoV-2 proteins.11,12 The tissue dysfunction caused by these apoptotic cells might exacerbate nearby inflammation. Several published studies show that individuals with critical COVID-19 symptoms are more likely prone to having dysfunctions related to the neural systems, such as encephalopathy, acute cerebrovascular illness, disturbances, cognizance, significant agitation and disorientation, corticospinal tract symptoms, or ischemic strokes. Some individuals solely exhibit neurological complications such as headaches, weariness, uneasiness, and evidence of hemorrhage or infarction in the cerebral cortex. Epileptic seizures, encephalitis, necrotizing hemorrhagic encephalopathy, strokes, and rhabdomyolysis have also been testified in association with COVID-19 infection.15–17 In many patients, some long-term after-effects have also been identified and reported, namely, a significant musculoskeletal load, including ligament, tendon, bone, and joint abnormalities, which can be included under the bracket of musculoskeletal and neuromuscular dysfunction.18
The outbreak of the novel coronavirus disease has reshaped our understanding of infectious diseases and their effects on human health. Initially characterized by respiratory symptoms, COVID-19 has an impact on various physiological systems. While the primary focus of research and clinical attention has been on the respiratory manifestations of the disease, recent evidence has highlighted the involvement of the musculoskeletal and neuromuscular systems in both acute and long-term phases of the illness. By addressing the intricate interplay between the SARS-CoV-2 virus and the musculoskeletal and neuromuscular systems, this comprehensive review aims to contribute to the evolving knowledge landscape of COVID-19 and its consequences beyond respiratory infections.
Musculoskeletal and neuromuscular dysfunction in COVID-19Musculoskeletal dysfunction (MSD) is a disorder associated with the musculoskeletal system and affects the body movements and locomotion of the concerned human being.19 Bones, ligaments, tendons, blood vessels, discs, nerves, etc., are the components of the musculoskeletal system. A survey of middle-aged individuals who have recuperated from COVID-19 infection shows that several symptoms of musculoskeletal dysfunction are reported in them (Table 1). The most severe indications of MSD seen in SARS-CoV-2 infected persons remain to be myalgia, arthralgia, fatigue, and inflammation. Myalgia is the most prominent clinical characteristic of COVID-19. Additional musculoskeletal expressions of the virus were recorded seldom early in the pandemic. The assessment of musculoskeletal symptoms and iatrogenic effects stemming from COVID-19 can be effectively conducted through various diagnostic modalities, including Computed Tomography (CT), Magnetic Resonance Imaging (MRI), and ultrasound. These advanced imaging techniques play a crucial role in detecting, characterizing, and evaluating the range of musculoskeletal and neuromuscular issues arising from COVID-19, as well as those resulting from medical interventions.
Musculoskeletal diseases associated with SARS-COV-2 infection.
S. No. | Musculoskeletal diseases | Risk associated | |
---|---|---|---|
1 | Myositis, polymyositis | Moderate to high | 20 |
2 | Duchenne muscular dystrophy | High | |
3 | Myotonic dystrophy | Moderate | |
4 | Congenital myopathy | Moderate to high | |
5 | Limb-Girdle muscular dystrophies | Moderate to high |
The COVID-19 pandemic has been linked to a wide variety of neuromuscular disorders, and it has been seen that SARS-CoV-2 also has direct or indirect effects affecting the neural tissues and thus cause diseases involving the brain and nerves have been reported in symptomatic as well as asymptomatic individuals.16,21 Acute cerebrovascular illnesses, disturbance in alertness, encephalopathy, significant anxiety and disorientation, ischemic strokes, or corticospinal tract symptoms are some of the reported problems in such patients. Some individuals solely exhibit neurological symptoms such as headache, weariness, uneasiness, and evidence of cerebral hemorrhage or infarction. Encephalitis, necrotizing hemorrhagic encephalopathy, strokes, epileptic captures, and rhabdomyolysis have also been testified in SARS-CoV-2 infection.15,17 Focussing on these effects (Table 2), the World Federation of Neurology has emphasized on reporting and registering the short-tenure and mild and long-tenure and critical results of COVID-19 on the neuromuscular system.22 These disorders are a collection of extremely different ailments, most of which are hereditary or autoimmune in nature and can affect individuals of all age groups at varying rates. A few publications on the subject have recently been published, emphasizing the importance of clinical research in developing confirmation-based guidelines to reduce death owing to COVID-19 in patients with neuromuscular problems.16 The hazards for these individuals, as outlined in a comprehensive study by Guidon A and Amato A, are dependent on various aspects, including the neuromuscular conditions, other comorbidities, age, and the kind of immunotherapy they get.23
Neuromuscular diseases associated with COVID-19.
Neuromuscular diseases | Risk associated | |
---|---|---|
Inherited neuropathies | No risk to simple Charcot–Marie-Tooth. Highly risky in individuals with neuromuscular chest disorders | 20 |
Multifocal motor neuropathy | Intravenous immunoglobulin is unlikely to increase risk. Cyclophosphamide is a considerable danger. | |
Guillain-Barre syndrome | Only when severely ventilated and with neuromuscular respiratory impairment are you in danger. There is no greater risk of causation or recurrence. | |
Chronic inflammatory demyelinating polyneuropathy | Risky in individuals with diaphragmatic problems. |
A phenomenon known as “cytokine storm” is characterized by an intense inflammatory response owing to the generation of a large count of pro-inflammatory cytokines, which is related to COVID-19.24 Cytokine profiles analysis of several COVID patients suggests that the phenomenon of cytokine storm is directly related to health problems such as multiorgan failure, musculoskeletal dysfunction, and neuromuscular dysfunction. Cytokine Storm Syndrome (CSS) has also been linked with the many reported deaths due to COVID-19.25
Cytokines are a major player in the inflammatory process. Several cells of the immune system generate cytokines (Fig. 1). The raiding virus generates an innate immune response in the body, which is detected by the PRRs (Pattern Recognition Receptors), which have the capability of sensing unique molecular arrangements and structures present on any foreign body. Pathogen-associated molecular patterns (PAMPs) are the names given to these molecular structures. When PRRs bind to PAMPs, they initiate the response of inflammation against the virion. Transcription factors are released when different signaling pathways get activated, which expresses genes encoding pro-inflammatory proteins that help in fighting against the virus.26
ACE2 and TMPRSS2 receptors: Viral entry points and beyondACE2 is present in epithelial cells of alveoli. SARS-CoV-2 takes the assistance of TMPRSS2 to bind to them.27 Type I interferon (IFN1) gets suppressed by viral components (nsp6, ORF6) when it blocks the translocation of IRF3 into nucleus. Actions of IFN1 responses get suppressed by pre-existing type I interferon autoantibodies targeting IFN 2 that suppresses signals from the IFNAR1–2 complex. Late response of IFN1 leads to the synthesis of proinflammatory chemokines and cytokines, which attract T cells and monocyte-derived macrophages to the lungs.28 Proinflammation signals by active macrophages recruit neutrophils into the lungs, where they undergo NETosis, causing necrosis. The CD4+ Th1 cells allocated into the lungs promote an inflammatory macrophage through IFN-signaling. In critical infection conditions, immune responses involve B cell activation to produce antibodies. However, inadequate antibody titer and formation of autoantibodies lead to unstable and imbalanced immune reaction, which causes hyper-inflammation, a “cytokine storm,” “Acute Respiratory Distress Syndrome” (ARDS), and, in numerous cases, death.29
It is thought that SARS-CoV-2 attacks type-II pneumocytes lining the respiratory passage, having ACE2 and TMPRSS2 during the early respiratory infection. COVID-19 is primarily a respiratory illness that can progress in a variety of ways, from remaining asymptomatic to moderate upper respiratory tract infection symptoms to ARDS.30 Extra-pulmonary signs of coronavirus infection are now known to include gastrointestinal symptoms, renal and liver damage, cardiac dysfunction, acute coronary syndromes, neuronal sequelae, and dermatologic abnormalities. To determine if musculoskeletal tissues express TMPRSS2 and ACE2, formerly published human genetic sequencing data were analyzed (Table 3).13
Expression of ACE2 and TMPRSS2 by various cells.
Tissues | Receptors | Cells | References |
---|---|---|---|
Respiratory air epithelium | TMPRSS2 and ACE2 | B and T lymphocytes, macrophage and mast cell, Type 1 and 2 alveoli cell | 31 |
Human skeletal muscle | TMPRSS2 | Pericytes, endothelial, smooth muscle, satellite cells, macrophage, and myonuclei | 32 |
ACE2 | Cells of smooth muscle and pericytes | ||
Cells of synovium | TMPRSS2 and ACE2 | Monocytes, fibroblasts, B and T lymphocytes | 33 |
Articular cartilage | TMPRSS2 | Homeostatic chondrocytes | 34 |
ACE2 | Proliferative, hypertrophic, and effector chondrocytes | ||
MENISCUS | TMPRSS2 | Not detected | 35 |
ACE2 | Progenitor cells of cartilage and regulatory fibro chondrocytes | ||
BRAIN | TMPRSS2 and ACE2 | Pericytes, smooth muscle cells in the vascular wall, choroid plexus, and neocortical neurons. | 36 |
The data point to skeletal muscle, cortical bone, and synovium as possible sites of SARS-CoV-2 contagion. SARS-CoV-2 may create a “cytokine storm” after binding to ACE2 receptors, with notifiable increases in IL-1, IL-6, and TNF levels. Extreme doses of the cytokines mentioned above promote vascular permeability, edema, and extensive inflammation, causing injury to several parts of the body. The cytokine storm syndrome (CSS) also sets off hypercoagulation cascades, which result in minute and big blood clots. ARDS, renal dysfunction and failure, liver injury, heart failure, myocardial infarction, and a variety of diseases related to neurons are all caused by the combined hyperactivation of inflammatory markers, vascular injury, and coagulation factors.3,10 Other coronaviruses have been shown to take entry into the brain directly, which may pave a path in COVID-19's potential input in demyelination or neurodegeneration. Likewise, SARS-CoV-2-activated cytokines can cause vasculitis in and around nerves and muscles, defined as “cross-reactivity” of antibodies (produced in reaction to viral antigens) with particular proteins on the myelin sheath, axon, or neuromuscular junction. While the direct invasion of virions into peripheral nerves is a potential scenario, there is also a viable hypothesis suggesting that immune-mediated mechanisms could be responsible for peripheral and cranial neuropathies, as well as muscle injury, in individuals infected with SARS-CoV-2.
Entry route and mode of musculoskeletal and neuro-invasionMusclesMyalgia, which is characterized as muscular twinges and soreness, has been recorded often in COVID-19-infected individuals, having a frequency range of 11%–50% in major cohort readings.37,38 Rhabdomyolysis is a severe infection that leads to acute renal dysfunction, internal bleeding, tissue swelling, and intravascular coagulation. Myalgia/feebleness and increased levels of creatine phosphokinase are 2 clinical symptoms of myositis/rhabdomyolysis detected in COVID-19 individuals.39,40 Rhabdomyolysis and myositis have been recorded in numerous case reports in COVID-19 individuals, both as a delayed consequence and as a presenting sign.41,42 There have been a few reports of SARS-CoV-2 causing necrotizing autoimmune myositis.43 In a study of 214 patients who have been hospitalized due to COVID-19 in Wuhan, 19% had the enzyme creatine kinase (CK) values of more than 200 U/L (a frequently used criterion for clinically high CK), with a maximum limit of 12 216 U/L. Up to a fair percentage of patients (36%) experienced vague neurological symptoms affecting motor coordination and, hence, muscular function. SARS patients have also been observed to develop severe myalgias and muscular weakness.44 The average CK level in individuals showing symptoms of moderate SARS-CoV-2 infection was 269 U/L, while it reached 609 U/L in the ones with severe SARS. Patients with critical SARS experienced a 32% drop in handgrip strength, and the capacity of distance walking reduced by 13% during a time period of 6 min when compared to people of the same age with healthy controls roughly 60–80 days after release from the isolated condition.37,45–47
Dysfunction in the muscle of the diaphragm in patients can arise due to critical illness myopathy, damage in the phrenic nerve, or ventilator-induced diaphragm dysfunction, potentially as a result of chest support device implantation.48 Neuromuscular effects of the SARS-CoV-2 may, in theory, lead to diaphragm muscular dysfunction. In fact, a recent study on autopsy investigation discovered the expression of ACE2 in the human diaphragm muscle of a subgroup of infected and controlled ICU patients. Evidence of SARS-CoV-2 viral RNA was detected in the diaphragm of patients, indicating viral presence within myofibers. RNA sequencing analysis revealed that fibrosis-related pathways, particularly fibroblast growth factor signaling, were activated. This was consistent with increased epimysial and perimysial fibrosis, which was more than 2 times higher in COVID-19 ICU patients' diaphragms compared to controls.49 Dysfunction of the diaphragm can worsen respiratory health and/or raise difficulties, which forces one to take up artificial ventilation support.
Sarcopenia and cachexia have been defined as long-term muscle sequelae in COVID-19 patients with severity.50 Sarcopenia (also known as myopenia) is characterized as muscle loss caused by age; other factors such as inactivity and poor diet can also contribute. Cachexia is muscular wasting caused by a persistent disease. Muscle atrophy, as observed in myopenia and cachexia, is characterized by reduced muscle size and fat infiltration on MR imaging.51,52
Mechanism of infectionThe mechanism by which the infection is creating its effects on skeletal muscles needs to be better understood. Within 4 days of infection, a mouse model of SARS experienced a 20% decrease in body mass.53 Several small studies have used postmortem reports of muscle tissue collected from deceased SARS patients to gain knowledge about the nature and degree of muscle dysfunction caused by SARS-CoV-2 infection.54,55 Muscular tissue and muscle fiber atrophy was widespread, with erratic and focal necrosis and infiltration of cells of immune system.55 Studies of the reports of electron micrographs showed myofibril hysteria and Z disc streaming,54 both of which impede forced transmission and have been observed in other muscle disorders.56,57 SARS patients have also been reported to have demyelination of neurons,58 which may donate to muscle faintness and fatigue. This can ultimately lead to stroke.59 These pathological alterations in the tissue skeletal system may occur due to the pro-inflammatory factors released due to the CSS caused due to viral invasion. CRP (C-reactive protein) is a standard inflammation biomarker, and various reports have shown that the level of CRP in severely ill COVID-19 patients is several fold-higher than normal values.60–63 Several pro-inflammatory signaling molecules that are known to be amplified in COVID-19 individuals can also have an effect on skeletal muscle. IFN-ϒ, IL-1, IL-6, IL-17, and TNF-α have the ability to directly promote muscle fiber proteolysis and reduce translation (Fig. 2).64–67 Certain progenitor cells, called satellite cells, contribute directly to muscle fiber development, which is critical when patients get recovered from COVID-19,68 and IL-6, IL-1β, and TNF-α may inhibit their propagation and variation.69,70 IL-1 and IL-6 can stimulate muscle fibroblast commotion to cause fibrosis, that can impede muscular power generation and make it more vulnerable to damage.71 Furthermore, corticosteroids were widely utilized to reduce acute inflammation in SARS patients, and these medicines can directly produce muscular atrophy and weakening.72–74 However, the Centers for Disease Control and Prevention (CDC) counsels against recurrent corticosteroid usage in COVID-19; therefore, corticosteroid-induced muscular weakness may have a smaller role in COVID-19 recovery.
Anticipated mechanistic pathway of SARS-CoV-2 musculoskeletal invasion.
(i) The virus infects the receptors, ACE2 and TMPRSS2, of the respiratory epithelium, which causes pulmonary inflammation. Cytokines released as a result of systemic inflammation reach the skeletal tissues via systemic circulation and cause infection. (ii and iii) ACE2 and TMPRSS2 receptors are also present in skeletal muscle tissues and cells of synovium, which act as potent receptors for the viral particles.
There have been multiple instances of peripheral neuropathies arising as a consequence of severe COVID-19, including compressive neuropathy, problems of the mixed central and peripheral nervous system, symmetric polyneuropathy, and systemic consequences from precarious illness neuropathy.75 In addition, proteins of the SARS-CoV-2 virus were reported in the medulla and cranial nerves IX and X in studies of autopsy series of COVID-19 patients.76 SARS-CoV-2 CNS attack has been denoted by similarity with the neurotropic syndromes of other coronaviruses, primarily MERS-CoV, SARS-CoV-1, and OC43.77 The possibility of SARS-CoV-2 acting as a novel pathogen of neural system and directly invading peripheral neurons via the ACE2 receptor merits auxiliary research. Given the resemblances among SARS-CoV-2 surface glycoconjugates and glycoproteins in human neurological tissues, the idea of “molecular mimicry” is an alternate explanation that may explain outlying nerve damage.
Mode of invasionStudying the different routes of entry of coronavirus into the body could lead us to the gateway of the mechanism of infection of the pathogen. Depending upon the infectious mechanisms of the former coronaviruses, numerous possible routes for the entry of SARS-CoV-2 have been proposed (Fig. 3).77
Viral particles attach to the ACE2 and TMPRSS2 receptors on the mucosal epithelium of the respiratory pathway. These particles then enter the systemic circulation, which leads to an immunogenic response and causes a cytokine storm. The cytokines bind to the endothelium of BBB and increase its permeability, which causes infection in the brain.
Other routesOlfactory routeOne of the first indications of COVID-19 infection has been observed to be anosmia. It has been verified with proof that there is an upsurge in MRI signal to the olfactory cortex, which is evocative of a neurological illness.78 ACE-2 receptors in the mucosal lining of the nasal chamber and neural tissues are thought to be the cause of the viral infection's neurological symptoms.79 The general circulation serves as a gateway for the viral entry into the neural blood flow. The slow rate of blood microcirculation, in association with the fast spreading of contagion from the primary sites of contamination, expedites the potent interaction of spike (S) protein and ACE-2 present in the endothelium. Subsequent to this, the viral budding in the endothelium causes the virus to spread in the brain and brainstem by passing through Virchow-Robin gaps, which are surrounded by arterioles and venules (Table 4).
Mode of infection of COVID-19 to different sections of the nervous system when infected through the olfactory route.
Sections of the nervous system | Mode of infection | References |
---|---|---|
Central nervous system | Through the circulation, infecting endothelial cells | 19 |
Peripheral nervous system | Via retrograde neural pathways | |
Other areas of the brain | Absorbed in nerve terminals, transmitted retrogradely, and spread trans-synaptically | 80 |
The arteries and veins that vascularize the CNS create a protective barrier called blood–brain barrier (BBB), which allows selective efflux and the influx of biomolecules and other solutes present in the blood into the brain.81 Despite being so protective, the BBB is a typical pathway for blood-borne viruses to enter the brain.77 COVID-19 viral spread into the blood has been documented, albeit at varying rates (1%–41%), and the viral particles take entry into the brain.82 Prevalent comorbidities in COVID-19 include vulnerability to cardiovascular diseases and neurological conditions that can potentially increase the permeability of the BBB alone or in conjunction with cytokines. Comorbidities common in COVID-19 possess the risk of cardiovascular diseases or pre-existing neurological ailments, which could enhance BBB permeability alone or in conjunction with cytokines.83 For example, electron imaging detected viral particles in the micro-vessels of the frontal lobe of the brain and neurons in an infected patient with Parkinson's condition, indicating the trans-endothelial entrance.84 The autopsy report of a patient who had obesity, hypertension, and diabetes and died from Parkinson's disease was studied. It showed the patient had micro-hemorrhages, white matter lesions, and hypoxic–ischemic neuronal damage. However, no traces of SARS-CoV-2 were found within the brain.85,86 SARS-CoV-2-associated cytokines, on the other hand, such as IL-6, IL-1, TNF, and IL-17, disrupt the blood–brain barrier, which may enable viral entry (Fig. 4).83 Although COVID-19 has been linked to the cause of inflammation in peripheral arteries and endothelial infection,87 no direct evidence of infection in brain endothelial cells has been found. Rather, numerous autopsy investigations have found with a lack of florid cerebrovascular infection.77,88,89 Virus particles can infiltrate the brain via infected immune cells90 and enter the brain via the vascular tissues, choroid plexus, and meninges,91 which might serve as entrance pathways for infected cells of the immune system. But yet, no irrefutable proof of SARS-CoV-2 contagion of immune cells has been reported.92 The presence of SARS-CoV-2N-protein immunoreactivity was documented in CD68+ macrophages, a cell type found within lymphoid organs. This observation was substantiated by the identification of viral RNA genomes within macrophages from the broncho-alveolar lavage of COVID-19 patients, as confirmed through single-cell RNA sequencing.93 It is unclear whether this is because of viral proliferation in macrophages, the phagocytic preoccupation of virus-infected cells, or extracellular virions.85,92 In addition, some autopsy statistics have demonstrated a significant paucity of infiltration of immune cells.89
Inflammation and opening of BBB are influenced by the circulating viruses, cytokines, DAMPs, and PAMPs, which are capable of acting on endothelial cells. Once in the perivascular region, these substances have the potential to cause inflammation in brain-resident myeloid cells (microglia and macrophages) and vascular mural cells. The ensuing cytokine secretion might impair brain function, resulting in “cytokine sickness,” a probable reason behind encephalopathy in COVID-19.
Evaluation of COVID-19 vaccine safety for patients suffering from musculoskeletal and neuromuscular disordersSignificant steps have been taken to create effective vaccines against COVID-19, which are being considered by a wide pool of possible candidates. The process of development has been sped up significantly, in contrast to the customary prolonged time frame. There are now a variety of vaccination candidates being tested in preclinical phases. These candidates cover a wide range of kinds. Notably, there are no plans to develop any live viral vaccines, which is a crucial concern for individuals with impaired immune systems who are required to avoid live vaccinations. More research is needed to determine whether or not immunosuppressive or immunomodulatory medications have an influence on the efficacy of COVID-19 vaccinations. This is especially important to know for those who have neuromuscular and musculoskeletal dysfunctions.
A study by Machado et al.94 aimed to assess the safety of SARS-CoV-2 vaccines in individuals with inflammatory/autoimmune rheumatic and musculoskeletal diseases. Most participants received the Pfizer/BioNTech vaccine, followed by AstraZeneca/Oxford and Moderna95. Among fully vaccinated individuals, breakthrough infections were rare, occurring in 0.7% of inflammatory autoimmune rheumatic and musculoskeletal diseases (I-RMD) patients and 1.1% of non-inflammatory- autoimmune rheumatic and musculoskeletal diseases (NI-RMDs) patients. I-RMD flares were reported in 4.4% of cases, with 0.6% being severe and 1.5% resulting in medication changes. Adverse events were reported in 37% of cases, with serious adverse events being rare (0.5%). The study concluded that SARS-CoV-2 vaccine safety profiles in I-RMD patients were reassuring and comparable to those with NI-RMDs, with most patients tolerating the vaccines well.
Conclusion and Forthcoming StandpointsThe understanding of various processes that cause extra-pulmonary disorders due to COVID-19 infection and the mode of infection of the virus can help physicians and scientists in designing a therapy modality that takes into account the urgent status of COVID-19 patients. Knowing the molecules and mechanisms that cause cytokine storms can help to use glucocorticoids, which have an anti-inflammatory effect and can help in the treatment of COVID-19 by inhibiting the pro-inflammatory actions of cytokines. Knowledge of the working behavior of the receptors ACE2 and TMPRSS2 can help scientists study their inhibitory mechanism and, accordingly, design drugs and molecules to inhibit the binding of the viral proteins to the receptors. Therefore, it becomes very important to specifically identify the inflammatory pathways and therapeutic targets of COVID-19. Although in the present day, there exist many therapies and vaccines for the treatment of the disease, more studies must be carried out to find treatments that act more efficiently against the deadly disease.
However, the anticipated data from neuromuscular disorder-specific organizations over this time period, outlining the risk factors and results of COVID-19 among these patient groups, will play a very important role in the formation of future risk stratification techniques. When the vaccinations become available, it will be necessary to think about the practicalities of vaccinating people who have neuromuscular illnesses. This is particularly true for people who receive medicine or who engage in self-isolation as a kind of medical treatment.