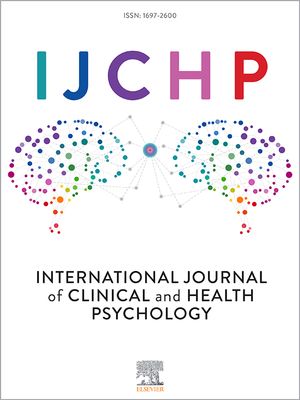
Edited by: Óscar F. Gonçalves
More infoIt was with the promise of rendering an experimental approach to consciousness that psychology started its trajectory as an independent science more than 150 years ago. Here, we will posit that the neurosciences were instrumental in leading psychology to resume the study of consciousness by projecting an empirical agenda for the future. First, we will start by showing how scientists were able to venture into the consciousness of supposedly unconscious patients, opening the door for the identification of important neural correlates of distinct consciousness states. Then, we will describe how different technological advances and elegant experimental paradigms helped in establishing important neuronal correlates of global consciousness (i.e., being conscious at all), perceptual consciousness (i.e., being conscious of something), and self-consciousness (i.e., being conscious of itself). Finally, we will illustrate how the study of complex consciousness experiences may contribute to the clarification of the mechanisms associated with global consciousness, the relationship between perceptual and self-consciousness, and the interface among distinct self-consciousness domains. In closing, we will elaborate on the road ahead of us for re-establishing psychology as a science of consciousness.
Imagine you are among a restless crowd of health professionals zigzagging around the ICU high-tech paraphernalia. In front of you, a patient who went into a coma after a cardiac arrest seems oblivious to any sights and sounds. His-eyes are open but revolving errantly around the room. No purposeful tracking, no intentional fixations, and no response whatsoever to any of your communication attempts. Although every evidence points to an absolute lack of consciousness, you cannot stop wondering if there is not any residual world of subjective experience, a “waking” mind locked in an unresponsive body.
Moving now from the bed to the bench, picture yourself in a quieter setting, but still surrounded by the familiar high-tech bio-labs paraphernalia. This time, instead of a patient, you stand before a series of images fed by a sophisticated microscope, allowing you to witness the assembling of a “brain”. Starting all the way from human somatic cells, then cleverly reprogrammed into pluripotent stem cells, through the use of the Yamanaka factors, diligent young researchers are doing the magic of building three-dimensional structures that model cells and tissue differentiation of multiple brain regions and circuits. While witnessing the reconstructed images of these “mini-brains”, in a structural form known as an organoid, again, you cannot stop questioning yourself if we are not fathoming the early inception of an “in-vitro consciousness”.
Time to move now to this immense storage house, where a blend of lab coats and coveralls interact over robotic apparatuses, virtual reality devices and artificial intelligence algorithms. Their engineered outputs are increasingly sophisticated, ever more interactive and flexible. Whether in the configuration of a personal assistant, a domestic cleaner or a wheelchair, it is difficult to keep from attributing an anima to these otherwise mechanical devices. No more easy bets at separating Anima from Machina. In this less aseptic industrial-like context, for a short while, you cannot stop wondering if we are not in the verges of an “in-silico consciousness”.
These examples, while hypothetical, illustrate circumstances that are very much real and unfolding as we speak. Thus, with the increasing plausibility of human “covert consciousness” (e.g., seemingly unconscious patients), “in-vitro consciousness” (e.g., laboratory-grown brain systems) and “in-silico consciousness” (e.g., artificial intelligence), it has become more urgent than ever to investigate these possibilities, if anything for their ethical implications. But how well-equipped is contemporary consciousness science to effectively address these questions?
It was with the promise of rendering an experimental approach to consciousness that psychology started its trajectory as an independent science more than a century ago. As ably captured by Chalmers, philosophers are “science incubators” (Chalmers, 2022). Theirs is the job of raising questions, and as those questions get addressed with increasingly rigorous methods, sciences start to emerge. The first experimental psychologists (such as Whihelm Wundt or William James) brought consciousness to the forefront by seeking to understand how the physical world is perceived by the individual (i.e., consciously experienced). In the 19th century scientists began to hypothesize that there could be a conscious and unconscious aspect of the mind, and that our cerebral cortex was involved in this process (Ferrier, 1877). Later, from 1950 onwards, researchers started grasping visual consciousness through studies of patients with amnesia, split brain and blindsight, since there was a clear dissociation between what they could perform behaviorally and what they could consciously report (c.f., LeDoux et al., 2020).
By the turn of the 21st century, researchers were joining in interdisciplinary efforts to identify the neural correlates of consciousness. Interestingly, the enterprise was led by Nobel laureate Francis Crick (Crick & Koch, 2003), who dreamt of decoding consciousness using a similar approach to the one he used a few decades earlier to decode DNA structure – by searching for the minimum neural mechanisms sufficient for conscious perception (Koch et al., 2016). This approach addresses, in essence, what Antonio Damásio recently referred to as the “intriguing pirouette” — how does the body provide us the mental experience of being ourselves? (Damasio, 2021b).
The current reviewHere, we will provide a synthesis of the contributions of psychological neuroscience to consciousness research, positing that the field of psychology was instrumental in resuming the study of consciousness and projecting an empirical agenda for the future (LeDoux et al., 2020).
We start by clarifying some of the most important conceptions associated with consciousness. Then, we will show how neuroscientists were able to venture into the consciousness of supposedly unconscious patients, opening the door for the identification of important neural correlates of distinct states of “covert” consciousness. This will be followed by a discussion of how different technological advances and elegant experimental paradigms were fundamental in establishing neuronal correlates of global consciousness (i.e., being conscious at all), perceptual consciousness (i.e., being conscious of something), and self-consciousness (i.e., being conscious of oneself). We will then illustrate how the study of some complex consciousness experiences may contribute to the clarification of the mechanisms associated with global consciousness, the relationship between perceptual and self-consciousness, and the interface among distinct self-consciousness domains. In closing, we will elaborate on the road ahead of us for establishing psychology as a science of consciousness (Michel et al., 2019).
We believe that this empirically derived multidimensional approach will be instrumental not only in reconciling different theories of consciousness but, more importantly, in aiding the development of various methods and technologies to address the different dimensions of consciousness phenomena.
The variety of conscious experienceColloquially, we may think of being conscious as synonymous with being awake. If that was the case, our scientific enterprise would be considerably easier, as conscious experience would be regressed to an all-or-nothing condition comprised of two states – being awake or being asleep, with nothing in between. For clinicians, this simple dichotomy would come in quite handy in classifying disorders of consciousness—you either have it (and you are conscious) or you do not (you are in a coma). However, colloquiality does not serve well the ethical demands of patients’ care, and thus clinicians have promptly conceded that conscious experience may have several degrees of qualification and quantification. And in doing so, they have become one of the main contributors to the never-ending process of disentangling consciousness (Bayne et al., 2017).
At first, because comatose patients usually start awakening while still unresponsive, those who did so were referred to as being in a “vegetative condition”. Then, realizing the complexity of this state, clinicians chose to rename it to “unresponsive wakefulness syndrome”. On the long way to recovery, they called the need to introduce a new transitory stage - “minimally consciousness state” (MCS). But just as the wheel was starting to move, MCS was decomposed in MCS- and MCS+, while still facing the need to further define an “emerging from MCS” condition.
Nevertheless, studying and classifying states of consciousness is not restricted to the clinical setting. One can go back to the laboratory and test equating consciousness with sleep-wake cycles in an experimental setting. Starting with sleep, you cannot avoid noticing how the apparent “unconsciousness” of deep NREM sleep contrasts with the intensive “conscious” experience of REM sleep. Two stages of consciousness during sleep would be reasonable and manageable. But then, like with disorders of consciousness, things get increasingly complex as you choose to probe into each of these stages (REM and NREM). Not surprisingly, you find here different degrees of consciousness along NREM sleep. Because you are a scientist and you love numbers, you decide to number them (stages 1, 2, 3, 4 and then, realizing you probably went too far, you cut off stage 4) (Berry et al., 2017). At least, the acknowledgment of REM as a homogenous consciousness state is well-established, right? Not quite, since microstates of tonic and phasic REM sleep may evoke different consciousness states (Simor et al., 2020). Not even in the awake condition we can say that humans are uniformly conscious. In fact, states of consciousness may be even more complex and diverse during the wake (Bayne et al., 2016). Here arises an important distinction between global and local states of consciousness (Bayne et al., 2016) that will be delineated in the next paragraphs.
Overall, this section illustrates how complex the study of consciousness becomes when we look at the various ways in which consciousness is manifested both in clinical and non-clinical contexts. One the one hand, on a purely quantitative level, consciousness seems as dicotomous as life itself (it's either present or absent, rather than unfolding in a continuum), with inquiries of ‘how conscious’ a seemingly conscious individual is being as practical as assessing ‘how alive’ might an autonomously breathing individual be. On the other hand, on a qualitative level, it's undeniable that conscious experience, when present, comes in different flavors and that the panoply of phenomenal aspects that compose each experience vary in both quantitative and qualitative terms. Taken together, information about the quantity and quality of subjective experience has guided researchers and clinicians alike, often implicitly, to characterize consciousness in terms of ‘levels’, ‘degrees’, ‘states’ and ‘conditions’. However, the informativeness of these categories and dimensions of consciousness is usually held back by the fact that ‘consciousness’, as a construct and as a phenomenon, has come to be understood, in recent decades, as too complex and multifaceted to be regarded as a single entity, rather than an ensemble of distinct (but interacting) processes.
So, to unpack this complexity, we suggest taking a divide and conquer approach to the study of consciousness, focusing on the mechanisms that allow any system (biological or not) to be: (1) conscious at all (i.e., global consciousness), (2) conscious of something (i.e., perceptual consciousness), and (3) conscious of itself (i.e., self-consciousness) (see Fig. 1). By doing so, it becomes feasible to methodically address the problem of consciousness, which, multidimensional in nature, will gradually succumb as the mechanisms of its constituent dimensions are discerned (Seth, 2021).
In the following sections, we will frame some of the most important advances in neuroscience research within each of these dimensions of consciousness. But first, we will start with the ingenious discovery that kickstarted the ever-growing process of disentangling consciousness.
Venture into the consciousness of supposedly unconscious patientsProbably the best illustration of what ended up being a true game changer in the experimental study of consciousness took place in 1993 in Cambridge UK. The clinical attention of a young neuropsychologist, Adrian Owen, was caught by the case of a patient named Kate, a 26-year-old woman who suffered an acute disseminated encephalomyelitis and lapsed into a coma state. As is often the case, a few weeks passed until she evolved to a condition in which she could keep her eyes open, as well as maintain sleep-wake cycles, despite not having any signs of consciousness. She was thought to be in what was referred to as a vegetative condition. So, Menon et al. (1998) undertook the challenging enterprise of functionally scanning Kate's brain so as to probe for the existence of any residual consciousness. Astonishingly, the presentation of images of familiar faces activated Kate's fusiform face area . On top of that, the unplanned exposure to the computer's screen saver, just before the face presentation, was responsible for the activation of the primary visual cortex. For the first time, researchers showed that the brain of a supposedly unconscious patient responded to external stimuli of different levels of complexity.
However, the question remained - was this evidence enough that Kate was conscious of these stimuli? In order to address this question, a few years later, Adrian Owen undertook an even bigger challenge with a similar unresponsive wakeful young woman, who had suffered a traumatic brain injury (Owen et al., 2006). The patient was first tested in a series of fMRI paradigms involving the presentation of spoken sentences (simple or ambiguous). Again, just like Kate, data showed that the patient was processing language in the expected brain regions, namely those associated with simple (middle and superior temporal gyrus) and ambiguous sentences (inferior frontal gyri). However, the ultimate test of conscious access was put forward when the authors introduced, in the fMRI paradigm, a cunning method aiming to establish functional communication with the patient. This involved analyzing brain response to commands, namely having the patient imagine she was playing tennis, contrasted with imagining visiting rooms around her house. Surprisingly, a patient otherwise deemed unconscious “responded” to these verbal commands with the activation of the expected brain regions also evident in healthy controls for those same conditions, specifically the supplementary motor region for tennis imagery, contrasting with increased parahippocampal, posterior parietal and premotor activity in response to the navigation command. With this, mental response to a verbal command (as assessed by activity in task-dependent brain regions) was intended to replace open motor response as the core “consciousness marker” in clinical settings (Owen, 2019).
The above examples, illustrative of the possibilities of reaching out to patient's consciousness, even when faced with limited introspective access, led to the definition of two additional states: covert cortical processing (the case of Kate, where the brain responds to passive paradigms such as observing faces, listening to music, hearing words or sentences) and cognitive motor dissociation (the case of the second young woman, that showed brain responses to active paradigms such as a command to perform a mental task).
Still, while brain response to active paradigms is reliable evidence of consciousness (i.e., cognitive motor dissociation), it is still under debate if brain response to passive paradigms is evidence of covert consciousness. In any case, for the last two decades, even more sophisticated experimental paradigms have been trialed to tackle consciousness, either by using higher-level passive paradigms (e.g., brain's response to movies - Naci et al., 2014) or more complex active paradigms (e.g., instructions to communicate with brain response - Abdalmalak et al., 2020; Norton et al., 2023.
An immediate impact of this research has been the call for a new classification of nosological conditions for disorders of consciousness (Bayne et al., 2017; Edlow et al., 2021; Sanz et al., 2023) and the increased usage of science-based (e.g., functional neuroimaging) diagnostic methods (Kondziella et al., 2021; Sanz et al., 2021). This is of extreme importance since patients with covert consciousness are associated with a faster recovery of consciousness and an overall better prognosis (Sanz et al., 2021). Furthermore, since current clinical practice is ill-equipped to identify these patients, who are capable of willfully modulating their brain activity, a substantial fraction is erroneously deemed as fully unresponsive.
In sum, the findings of Owen and colleagues opened the door for the identification of important neural correlates of distinct states of “covert” consciousness. But most importantly, they showed that the validity of existing methods for identifying the presence of consciousness could be as elusive as the concept itself. In the next section, we will show how Integrated Information Theory (IIT) built on these findings to achieve a reliable method for identifying consciousness, in an ambitious attempt to conceptualize consciousness.
Neural correlates of global consciousness: integrated informationNo matter how relevant these neuroimaging markers are, they still depend on the individual's capacity to mentally respond to external stimulation or verbal commands, which is not often the case. No passive or active paradigms can be processed when structural lesions or cognitive impairments lead, for instance, to the absence of a preserved sensorial apparatus. However, it is not difficult to envision conditions in which an individual remains unresponsive to sensorial stimulation while still maintaining some sort of covert consciousness. This is, for instance, the case of REM sleep, in which the person has an elaborate oneiric experience while remaining mostly unresponsive to external stimulation (Lee et al., 2022).
The challenge would then be to find a way of probing the brain system in an attempt to assess global consciousness without resorting to sensorial stimulation (Massimini & Tononi, 2018). This may seem, at first sight, an insurmountable task. However, the proponents of one of the most ambitious theories of consciousness – the Integrated Information Theory (IIT) – were able to successfully respond to this demand with a combination of sound theory, ingenious technology, elegant mathematics and experimental control (Tononi et al., 2016).
Indeed, IIT departs from traditional scientific approaches to consciousness by proposing a fundamentally different epistemological framework. Instead of starting with observable physical phenomena and attempting to explain consciousness as an emergent property, IIT begins with axioms derived from subjective experience itself (i.e. essential properties of all conscious experience that are established as self-evident and thus accepted without necessity for proof), from which IIT derives postulates concerning the physical systems that could give rise to such properties of experience.
A brief theoretical explanation: as the name suggests, IIT posits that all conscious experience has two central features: it is both informative and integrated (Tononi et al., 2016). It is informative because every conscious experience is unique, differing from any past or future experiences, and it is integrated because every experience is always “one” (i.e., unified)– meaning that the multiple elements of experience are bound, and thus irreducible to non-interdependent parts. So, because we have, at any given moment, precisely one out of countless other possible conscious experiences, each experience becomes deeply informative – not only for what it is, but mainly for what it isn't (Seth, 2021). Ultimately, this information integration configures what cognitive scientists call reduction of uncertainty. In light of this, to be conscious is, first and foremost, to reach for information that reduces the experience of subjective uncertainty – “difference that makes a difference” (Oizumi et al., 2014).
Translating these theoretical claims into physical systems, the authors postulate that the same properties which permeate conscious experience – i.e., information and integration – should also dictate the functioning of its underlying physical mechanisms. More specifically, the theory claims that any physical system will be conscious to the extent that it can generate information as a whole, above and beyond the sum of the information generated by its individual components. This property is indexed by IIT as Φ, representing a quantitative measure of integrated information (Koch, 2019). Being conscious means, therefore, to be able to potentiate the balance between diversifying and integrating experience.
To illustrate this, imagine a model of a brain composed of a set of nodes (Fig. 2). In Model 1, the activity of every node is random and independent. Therefore, the system has the maximum amount of information (distinct activity at every node), but due to its random nature, and absence of interdependence between nodes, no integration is present (low levels of Φ). At the mind level, this would be translated into a chaotic stimulation flow without a coherent sense of subjective experience.
Moving to the other extreme, as represented in Model 2, all nodes seem to behave harmonically, in an absolute state of synchrony with no randomness. Such a system showcases absolute integration but the lowest amount of information power (configuring, once again, low levels of Φ). As for the mind, this would model a consciousness that integrates, but at the cost of foreclosing information (i.e., possessing a repertoire of just two states – on and off). Hypothetically, this would be translated into an unchanging and stereotypical subjective experience that, regardless of how the mind is stimulated, is always configured as that particular one (e.g. experience of a particular shade of red). Here again, we are in the presence of a restricted level of consciousness.
But there is still a third alternative, in which most of the nodes are active and generating diversified and flexible patterns of interaction (Model 3). At the mind level, we are modeling here a significant increase in consciousness, allowing a diversity of states that are functionally integrated (this time, configuring high levels of Φ), and thus a more coherent sense of subjective experience. Only here does the system reach what the study of phasic transitions in physics refers to as an “optimal state of network criticality”, a balance between order and disorder (both highly sensitive and highly integrative) (Kim & Lee, 2019).
Having laid out this theoretical framework, IIT was now tasked with its own empirical validation, which emerged as a twofold challenge. First, coming up with an empirical measure of Φ in brain systems (i.e., a valid and reliable method for gauging how much information is generated by a brain system that is above and beyond the information generated by its elements). Then, testing whether higher or lower levels of Φ in a brain system indeed marked the presence or lack of consciousness, respectively. Essentially, IIT needed to demonstrate that an empirical measure of Φ corresponded, in fact, to a “consciousness meter”.
To achieve the first step, a strategy was needed to introduce information into a “closed” brain system (not requiring any exteroceptive/interoceptive input) and assess how that information was being processed within the system (no motor output). IIT came up with an ingenious method (known as the “Zap-Zip”), in which transcranial magnetic stimulation is used to induce a perturbation in a brain system (zapping – introducing information in the form of electrical current), while electroencephalography (EEG) is simultaneously used to measure how this information is distributed and integrated within the brain's networks. To quantify these distribution/integration dynamics, the spatiotemporal EEG patterns are then compressed using the Lempel-Ziv-Welch algorithm (zipping – just as one would ‘zip’ a computer file), allowing for the estimation of the algorithmic complexity of the EEG signal. The magnitude of this complexity ultimately provides a quantified measure of brain complexity known as the Perturbation Complexity Index (PCI) (Casali et al., 2013; for PCI mathematical details, see Mayner et al. 2018). On paper, this method seemed to offer a solution to the challenge of coming up with an empirical approximation of Φ in brain systems.
But before moving to real brains, we will first illustrate how PCI can, in principle, be effective in modeling and detecting different consciousness states, starting with a simple simulation. Imagine again the previously mentioned Models 1, 2, and 3 (Fig. 2), and move them through the zap-zip method (see Fig. 3). Starting with Model 1, we induce a TMS on a given node, and as expected, we witness a TMS evoked potential just below the stimulation site. Here, the effects still spread to the nearby node, originating a weaker TMS-evoked potential. Both signals rapidly decay, though. In this model, the spatiotemporal patterns of the EEG signal remain very simple, thus requiring low compressing complexity. This would be translated into a low PCI, suggesting the system shows low levels of sensitivity and integration.
Moving now to Model 2, the perturbation induced in the same node not only evokes a response under the stimulation site, but it spreads uniformly with no differentiation across different sites and with a fast decay. Here, the system shows a maximum level of integration with minimum sensitivity. Again, the output signal requires low compressing complexity with a correlative low PCI index.
Finally, the third model simulates a state of high consciousness characterized by a maximum amount of sensitivity with optimal integration, and an optimal balance between order and disorder (i.e., system network criticality). Here, the perturbation induces the expected TMS-evoked potential under the stimulation site node, which subsequently spreads across other nodes and originates, as a result, distinct patterns of reverberant activity across time. Only here does the system express high PCI levels.
Moving now from models to real brains, it now seems plausible that PCI, when applied to individuals in different consciousness states (loosely corresponding to Models 1, 2, and 3), could provide the answer to the ultimate question of whether higher or lower levels of information integration in a brain system indeed mark the presence or lack of consciousness, respectively. We may start with the most basic question. Can the PCI algorithm successfully discriminate awake from deep sleep consciousness?
This is indeed the case, as PCI values are higher in the awake state and very low in the deep NREM sleep (Massimini et al., 2005). Additionally, PCI is able to distinguish REM from NREM sleep, with REM sleep evidencing PCI levels similar to awake consciousness (Massimini et al., 2010). PCI is also successful in discriminating wakefulness from unconscious states induced with general anesthesia (e.g., midazolam, propofol, xenon). Curiously, individuals under ketamine, a dissociative anesthetic known to induce intensive oneiric experiences, show PCI levels compatible with REM sleep state, or awake condition (Sarasso et al., 2015).
The final test of the model would also require a significant accuracy of PCI in classifying patients with distinct disorders of consciousness. Also here, it was possible to come up with a PCI threshold level that clearly distinguishes patients who are unconscious (Coma, Unresponsive Wakefulness Syndrome) from those who are conscious despite being unable to provide a motor response (Locked-in Syndrome) or are minimally conscious (Casarotto et al., 2016).
Bringing all this evidence together, a test of a large data set of individuals undergoing this procedure (subjects during wakefulness, REM and NREM sleep, under several anesthetic agents, and patients with different consciousness conditions) confirms PCI as a reliable measure in discriminating conscious from unconscious states (Casali et al., 2013).
While IIT remains a good illustration of how the joint efforts from psychology, neurosciences and technologies provide a new experimental window into global consciousness (i.e., neuronal correlates of “being conscious at all), IIT has not been spared controversy on conceptual, mathematical and methodological grounds. At the conceptual level, IIT's central assumption of equating consciousness with differentiated-integrated information has been under debate. And indeed, while PCI research has arguably established some of the most convincing explanatory relationships between neural dynamics (i.e. spatiotemporal brain complexity) and subjective experience, the extent to which PCI provides an empirical measure of Φ is still unclear. As recently remarked by Merker et al. (2022), IIT fails to show how the differentiation-integration dynamics constitute a necessary (let alone sufficient) condition for subjective experience (i.e., intrinsic and unique perspective). While trying to come up with a theory of how a system is globally conscious (i.e., how it is to be conscious at all), IIT may have failed in providing an explanatory model to understand a core quality of consciousness – intrinsic, unique and subjective experience.
In particular, at the mathematical level, the proposal of computing consciousness using an index of global information transfer increases the risk of further moving IIT away from addressing consciousness in terms of subjective experience. Several non-conscious network systems are able to efficiently accomplish global information transfer similar to what IIT predicts for consciousness. These efficient network systems include a diversity of biological (e.g., metabolic, immune and gene regulation networks) and non-biological domains (e.g., transportation, telecommunication and computer networks). By equating consciousness with a measure of information transfer, IIT moves closely to panpsychism (i.e., some non-life entities are conscious) or, at least, proto panpsychism (i.e., some fundamental entities are proto conscious) (Chalmers, 2013). Relying on the mathematical solution advanced by IIT, it may very well be the case that other non-human systems (biological or non-biological) have global consciousness. As previously mentioned, IIT proposes that consciousness is not limited to complex organisms with sophisticated nervous systems, but is a basic feature present even in simpler systems and potentially even in non-organic systems. In this framework, all matter could have some form of consciousness or subjective experience. While this challenges the traditional understanding of consciousness, it currently lacks empirical evidence directly supporting the idea that all matter possesses some form of consciousness.
More recently, it has been attempted to sophisticate IIT's mathematical structure to take into account the qualia dimensions of consciousness (Kleiner & Tull, 2021). However, non-consistency has been found in the computation of simulated consciousness systems for different measures of integrated information (Mediano et al., 2019).
At the methodological level, while ingenious, the technological solutions proposed by IIT are time-consuming, expensive, and with limited sensitivity to discriminate dynamic fluctuations in both waking (e.g., mind wandering versus focus attention) and sleeping consciousness states (e.g., microstates within REM sleep). However, TMS-EEG acquisition (Caulfield et al., 2020), PCI computations (Comolatti et al., 2019), and experimental methods (Belardinelli et al., 2019) are being optimized and increasingly efficient machine learning algorithms being tested (Lee et al., 2022), with a promise of a wider applicability from the bench to the bedside. This would be of extreme importance for clinicians in the prognostication and treatment of severely brain-injured patients, since PCI can effectively and reliably measure a patient's capacity for conscious experience - covert or overt - without relying on a preserved sensorial apparatus or the patient's response.
In sum, while IIT has not yet provided a convincing explanatory model and empirical demonstration of some of the core ideas it initially set out to explain, it has, on the other hand, successfully operationalized explanatory causal relationships between brain complexity dynamics and global consciousness (i.e., how it is to be conscious at all). In addition, it established the theoretical-methodological foundations for an alternative neuroscience of consciousness that discerns the capacity for subjective experience not from what a system does (e.g. observable states), but from how it is done (i.e. how it is built) (Massimini & Tononi, 2018). This approach has proved useful in predicting which regions of the brain maximally contribute to global consciousness (a complex situated in the posterior cerebral cortex, in a temporo-parietaloccipital “hot zone”; Albantakis et al., 2023) and which do not (e.g. cerebellum, basal ganglia).
But ultimately, this approach seems ideally positioned to address whether in fact human “covert consciousness”, “in-vitro consciousness” and “in-silico consciousness” are indeed possible. The first has been demonstrated in previously mentioned investigations by Adrien Owen, with further confirmation by PCI studies. Regarding the second, it seems plausible that cerebral organoids, by mimicking the human brain's developmental processes of differentiation of brain regions and circuits, could eventually attain a level of architectural intricacy whereby the brain system will possess the intrinsic ability to influence itself, and thus sustain the capacity for conscious experience. The latter, on the other hand, while already instantiated in devices exhibiting human-like behavior, is predicted by IIT to be devoid of conscious experience as long as it processes information in purely feed-forwards networks (as is most common in machine learning algorithms) and does so virtually (i.e., software simulation). Still, Massimini and Tononi (2018) concede that if these forms of “in-silico consciousness” are physically based on hardware, which despite currently unfeasible could potentially become a reality in future evolutions of current neuromorphic circuits (e.g., Pfeil et al. 2013), and incorporate feedback loops or recurrent connections in information processing, “in-silico consciousness” could indeed emerge.
Neural correlates of perceptual consciousness: information availabilityWhile providing a good account of the neural correlates of global consciousness, IIT may fall short as an explanatory model of perceptual consciousness – how it is to be conscious of something. We may very well be awake and vigilant, showing high PCI values, but remain oblivious to some interoceptive and exteroceptive stimulation.
Therefore, in order to understand consciousness, we may need to go one step further, from “global” to “local consciousness” (Seth & Bayne, 2022) and try to untangle the mechanisms responsible for local (or perceptual) consciousness. In doing that, we need to call for another theoretical contender – the Global Neuronal Workspace (GNW) theory (Dehaene, 2014).
According to GNW, consciousness is, first and above all, about information availability. To understand consciousness, we need to study how information is made available to the whole organism, allowing individuals to report, recall and act upon it (Dehaene et al., 2011). GNW holds that consciousness depends on the existence of a neuronal workspace above and beyond the reticular-thalamic, thalamocortical and specific modular brain regions. This global neuronal workspace encompasses a network of widely distributed excitatory neurons (e.g., pyramidal cells from cortical layer II/III /V) that modulate (stimulate or inhibit) the incoming/outgoing functional connections to and from specific cortical modular regions (sensorial-perceptual, motor, memory, and evaluative information).
GNW hypothesizes three operational mechanisms: ignition, amplification, and broadcasting. First, information, being generated along bottom-up and/or top-down processes, when reaching a given threshold, causes a sudden non-linear ignition of the content-specific workspace neurons. Then, a set of re-entrant feedback loops between workspace neurons and specific cortical processors, produce signal amplification. Finally, the information is broadcasted to multiple cortical processes, allowing global availability of this information (the individual can name it, recall it, or act upon it) (Mashour et al., 2020).
Moving from theory to simulation, we can illustrate GNW's operational mechanisms using two contrasting models (see Fig. 4). Model 1 simulates the lack of conscious access in response to a brief and weak impulse. Here, we witness a feed-forward connection system in which the information propagates serially along a hierarchy of regions. In this model, the information fails to ignite the GNW and decays rapidly. Therefore, no conscious access is present in Model 1. Alternatively, in Model 2, an extended stronger impulse leads to re-entering loops of feedback projections. The feedback re-entrant system is responsible not only for the ignition and amplification of a long-lasting signal, but also for the global broadcasting of this signal to different cortical processors, allowing the information to be reported, recalled and acted upon. Model 2 simulates conscious access (Dehaene et al., 2003; Dehaene & Changeux, 2005).
It is time now to check if GNW still holds when applied to in vivo experimentation. Just like IIT, GNW faced the challenge of bringing its theoretical claims under the scrutiny of experimental psychology. A strategy was therefore delineated: the first step was to come up with an adequate strategy for manipulating contrasting perceptual awareness conditions, namely conscious versus unconscious processing. Fortunately, experimental psychology research had produced, over the decades, no shortage of paradigms that allow for maintaining a specific stimulus constant while contrasting the conditions of stimulus presentation (conscious versus unconscious perception), such as in “masking” (Bachmann, 2018) “binocular rivalry” (Carmel et al., 2010), “attentional blinking” (Ophir et al., 2020) or “continuous flash suppression paradigms” (Yang et al., 2014). A final experimental component to take into account was controlled introspection (i.e. a valid and reliable method identification of the presence of conscious perception). Even though some no-report paradigms are currently being tested (Block, 2019), for most of the strategies listed above, open reports on stimulus detection remains as the central criteria for conscious perception.
Confirmatory evidence for GNW theory was found in studies using a diversity of these paradigms. Specifically, conscious access signatures were identified for the neurophysiological mechanisms of ignition, amplification, and broadcasting (Dehaene et al., 2011; Mashour et al., 2020).
Based on this evidence, we will now illustrate how these mechanisms would unfold over the course of a visual masking paradigm. Using words as stimuli, this paradigm entails a task in which the presentation of masked words (e.g., words presented for 29 ms preceded by a forward mask and followed by a backward mask) is contrasted with a response to unmasked words (Gaillard et al., 2009). While both masked and unmasked words can reach from primary visual cortex to the low temporal visual word form area, only words that are consciously accessed make it to multimodal associate cortices, specifically igniting prefrontal and parietal regions.
So, if we were to record an EEG during the presentation of these stimuli, we would see that the electrophysiological signal starts to diverge after 200 ms., with the fading of the masked word contrasting with a significant amplification of the wave activity responsible for multimodal ignition in the case of unmasked words. This is evidenced by an increased amplitude of a positive wave around 300 ms - P3b wave.
Conscious access is thus not only illustrated by the ignition in multimodal brain activity, but also by signal amplification, as illustrated by the power increase of high frequency gamma activity after 200 ms for unmasked words. Even though masked stimuli can induce local transitory gamma activity, only with unmasked words for conscious processing can we identify a burst of high frequency brain activity that is generalized and sustained across multimodal brain regions, representing a signature of amplification.
Finally, even if the ignition of multimodal brain regions is already a precondition for signal broadcasting, the neurophysiological signature of global broadcasting is best illustrated by the increase in synchronic activity across distant brain regions. Effectively, and again after 200 ms, several populations of neurons synchronize in response to the presentation of unmasked words, which is particularly evident in the reciprocal communication among long distant nodes.
Some researchers have questioned the empirical evidence of these neural correlates of perceptual consciousness. For example, when comparing the neural signatures in report (i.e., participants instructed to report their perceptual experience) versus non-report masking paradigms (i.e., participants instructed to refrain from reporting their perceptual experience), it was found that the nonlinear increase in the P3b was only present in the report condition (Cohen et al., 2020). This data suggests that, by relying on introspective reporting paradigms, GNW theory may be tackling post-perceptual processing (e.g., working memory, decision making).
This configures a complex dilemma. While report paradigms risk targeting post-perceptual paradigms, no-report paradigms may be tackling unconscious processing (Schlicht, 2018). However, as argued by Damasio (2021b), while introspection has obvious limits, still no “rival” has given us a window into subjective experience, which is certainly at the core of perceptual consciousness. More recent formulations of the GNW have been trying to address some of these conflicting findings by means of computational modelling of conscious access (King & Dehaene, 2014; Whyte & Smith, 2021).
Finally, GNW is criticized for mainly addressing the functional aspects of the perceptual, with little to no attention to its phenomenological aspects (Seth & Bayne, 2022). Indeed, GNW research focuses mainly on identifying the process, rather than content-based neural correlates of consciousness (Marvan & Polák, 2020). In other words, GNW left out the central question of “how it is like to have a given perceptual experience?”. Therefore, in order to bring some light into this question, and bring a closure to our overview of consciousness research, we need now to move from “perceptual consciousness” to “self-consciousness”, the experience of being a self.
Neural correlates of self-consciousness: information locationWhile different, self-consciousness remains deeply intertwined with perceptual consciousness. Self-consciousness brings a self-perspective into perceptual consciousness. And it is this self-perspective that provides the “qualia” dimension to consciousness - “what is like to have a given perceptual experience” (LeDoux & Brown, 2017).
In discussing self-consciousness, we need to call for a final theoretical contribution – the model of consciousness as formulated by Antonio Damasio (Damasio, 2021b). In addressing the constitutive role of the self in consciousness, Damasio offers an important contribution. Paradoxically, despite the potential explanatory power of the model, the author seems resistant to formulating it as a “theory of consciousness”(Damasio, 2021a).
According to the author, an experience is rendered conscious once located inside a particular organism. This “self-location” is a constitutive feature of consciousness and not merely a variety of conscious experience. In addition, this model of consciousness holds that self-conscious organisms have four central features. First, the organism needs to have a nervous system for mapping perceptual information. Second, due to the lack of any clear border between brain and body, these perceptual maps are primarily interoceptive maps. The predominance of unmyelinated fibers, non-synaptic communication and gaps in the blood-brain barrier, bring the interoceptive system into more intimate contact with the rest of the body, constituting a central platform for body sensing and brain-body interaction (Carvalho & Damasio, 2021). Third, feelings, originating from these interoceptive maps, are responsible for bringing a self-perspective to perceptual experience (i.e., the “what-is-likeness” of having a given experience). Finally, the capacity to feel body states (i.e., interoceptive maps) - being the core of conscious experience - is further enhanced by contributions from exteroceptive, proprioceptive and memory processes. To a certain extent, the movement between perceptual consciousness and self-consciousness corresponds to a switch between exteroception and interoception (Denton, 2006).
Self-consciousness – defined as being “consciousness of oneself as oneself” (Milliere, 2020) - is thought to entail a mind (also referred to as “narrative”) and a body domain (alternatively called “core”). The mind domain is responsible for integrating subjective experience into a self-narrative. Complimentary, the body domain concerns the awareness of one's body as experienced through interoceptive (e.g., feeling hunger), exteroceptive (e.g., sensing back pressure), or proprioceptive states (e.g., arm movement).
Introspective and interoceptive states have been used to study the neural correlates of mind and body self-consciousness, respectively (Frewen et al., 2020). For example, in an fMRI experiment, Araujo et al. (2015) used a set of interoceptive (e.g., “Do you feel hungry?”) and exteroceptive probes (e.g., “Do your legs feel wet?”) to study a subject's access to body self-consciousness. Contrastingly, top-down introspective strategies were used in the same study to tackle mind self-consciousness, including personality traits (e.g., “Does the word honest describe you?”) and biographical information (e.g., “Are you a student?”).
Initial research on the neural correlates of self-consciousness, not distinguishing among domains (i.e., body and mind), found evidence for the role of the Default Mode Network (i.e., medial prefrontal/anterior cingulate and medial parietal/posterior cingulate cortical) in self-awareness access (Lou et al., 2017). However, as we look at different self-consciousness domains, a more complex picture starts emerging. While both domains (mind, body) entail the activation of common regions associated with memory (e.g., hippocampus) and body processing (e.g., somatosensorial processes), each domain is also associated with the activation of particular regions, with mind self-consciousness implicating core nodes of the DMN (e.g., anterior cortical midline structures), and body self-consciousness involving posterior cortical midline regions (Araujo et al., 2015).
The studies reported above, while important in bringing some initial support for the neural correlates of different self-consciousness domains, are still limited in terms of experimental control (e.g., over relying on self-report) and identification of causal mechanisms. Several authors have been trying to overcome these limitations by experimentally studying different aspects of body and mind self-consciousness. For example, a recent study with rhesus monkeys researched the effect of interoceptive awareness (heartbeat sensitivity) on different perceptual tasks (e.g., attention to heartbeat synchronous or asynchronous stimuli). Confirming results already found in human infants, the authors showed that also animals had increased attention when the stimuli were presented asynchronously with their heartbeat, illustrating the fact that exteroceptive processing was influenced by the integration of interoceptive stimulation (Charbonneau et al., 2022). Another recent investigation looked at the modulating effect of body awareness on mind self-consciousness by providing a first-person body view (i.e., virtual reality) during episodic memory encoding, showing that this modulation impacts the connectivity between networks associated with mind and body consciousness (Gauthier et al., 2020).
Additionally, at the conceptual level, the concepts of body and mind self-consciousness still need further clarification. For instance, some authors claim that, rather than being a single construct, body awareness may encompass several layers, from body schema to body image(Riva, 2014). Also, distinct sensorial (e.g., interoceptive versus exteroceptive) and brain mechanisms may be associated with different bodily self-consciousness domains (e.g. body identification versus body location) (Park & Blanke, 2019). The same may be true regarding mind self-consciousness, which may require further discriminations, for example, by disentangling the neuronal correlates of semantic versus episodic, perceptual versus conceptual or prospective versus retrospective self-consciousness (Palacio & Cardenas, 2019; Sheldon et al., 2019).
Summing up so far, specific markers were identified not only for discriminating conscious from unconscious conditions (global consciousness), but also for identifying signatures of perceptual consciousness. Additionally, there is now initial evidence of different brain signatures associated with different self-consciousness domains. Despite the increment of our knowledge on the neural correlates of all these different aspects of consciousness, there is a long way ahead of us to fully understand the relationship between global, perceptual and self-consciousness.
Neural correlates of complex consciousness experiencesAs discussed above, research has been fruitful in bringing an initial understanding of how any system is conscious at all (global consciousness), conscious of something (perceptual consciousness) and conscious of itself (self-consciousness). However, different consciousness phenomena may bring additional complexity to each one of these components, ranging from subtle alterations in attention to profound and transformative experiences. We refer to these alterations in the quality and intensity of the conscious phenomenology as “complex consciousness experiences” (known as “altered states of consciousness) (Chirico & Gaggioli, 2021). These include conditions such as, among others, mind wandering (Gonçalves et al., 2018), aesthetic absorption (Lange et al., 2017), awe (van Elk et al., 2019), flow (van der Linden et al., 2021), lucid dreaming (Baird et al., 2019), out-of-body (Blondiaux et al., 2021), near-death (Martial et al., 2020), or psychedelic experiences (Bayne & Carter, 2018).
Here we will briefly illustrate how the complex experiences of wandering consciousness, psychedelic (like) experiences and dying (like) experiences are now being used to bring further understanding to the neuronal correlates of consciousness, namely by providing useful research paradigms for understanding consciousness access, the relationship between perceptual and self-consciousness, and dissociation between mind and body self-consciousness, respectively.
Recently, we showed that the typical daily oscillation between focused and wandering consciousness is associated with the attenuation of some of the neurophysiological markers of consciousness access. Prior to reports of consciousness wandering, we found an attenuation of P3 wave, accompanied by an increase in the power of low frequency bands (theta, alpha) and a decrease in higher frequency bands (beta, gamma) (Dias da Silva et al., 2022). This suggests that some consciousness access markers may be present in different degrees for distinct awake conditions. While still able to consciously perceive the stimuli and perform an ongoing task, mind wandering may be an indicative of decreased task relevance. This is consistent with previous studies, which did not find the typical increase in gamma activity and the P3 for task irrelevant stimuli (Pitts et al., 2014).
Also, consciousness modulator drugs, such as classic psychedelics, are regaining new momentum in researching the neuronal correlates of consciousness, exploring conscious and unconscious information processing pathways between central and peripheral systems (Kelly et al., 2023), and untangling the perceptual and self-consciousness mechanisms (Hayes et al., 2020). Alterations induced by psychedelics include perceptual consciousness (intensification, alteration, novelty and synesthetic sensorial experiences) as well as self-consciousness (altered self-perception, self-dissolution) (Breeksema et al., 2020). While increased perceptual phenomenology was found to be associated with increased activity and functional connectivity in visual cortex, self-consciousness phenomenology (e.g., ego dissolution) was mostly associated with the disruption of functional connectivity within the core self-consciousness networks - DMN (Carhart-Harris et al., 2016). The phenomenon is well captured in the REBUS model (‘Relaxed Beliefs Under Psychedelics’) according to which the effects of psychedelics are dependent on the relaxation of self-consciousness systems by interfering with regular predictive processing of exteroceptive and interoceptive experiences (Carhart-Harris & Friston, 2019). The relaxation of self-consciousness systems may balance the system towards an intensification of incoming perceptual consciousness. While still in its initial stages, the use of psychedelics may be instrumental for a better understanding of the relationship between perceptual consciousness and self-consciousness. Likewise, the modeling of psychological and neural correlates of both perceptual consciousness and self-consciousness psychedelic like phenomena with virtual reality constitutes an important avenue for clarifying the relationship between “being conscious of something” and “being conscious of oneself” (Glowacki et al., 2022; Suzuki et al., 2017). Non invasive brain stimulation tecnhiques such as transcranial direct current stimulation (tDCS) have also been used to modify dimensions of consciousness such as self-awareness, namely with alterations of hypnotic experience after cathodal stimulation over the left dorsolateral prefrontal cortex (Perri & Di Filippo, 2023).
Finally, another complex consciousness experience is gaining the attention of researchers – Recalled Experiences of Death (known as Near Death Experiences) (Martial et al., 2020). Because of the developments of resuscitation science, an increased number of individuals are brought to life after being in a near death condition. A significant percentage of these patients report experiences that may suggest an increase in mind self-consciousness (e.g., life-review phenomena) along with a decrease in body self-consciousness (out-of-body experiences) (Parnia et al., 2022). Worth noting that recent research found evidence of brain activity suggestive of increased self-consciousness in a dying patient: EEG data recorded from a patient in transition to death showed an overall increase in gamma power after bilateral suppression of brain activity (Vicente et al., 2022). Curiously, an increase in functional connectivity within DMN was also found in end-of-life patients, suggesting that augmenting of mind self-consciousness may be the brain's response trade-off for a decreased awareness of a dying body (Blundon et al., 2022).
Wandering, psychedelic like and death like experiences are just three illustrations of the potential of looking at complex consciousness states as important research paradigms in consciousness research, be it on a microscale (neuron level), macroscale (brain regions) or from a psycho-phenomenological perspective (experiential profile).
The latter perspective, applied to complex consciousness experiences, has also gained traction in recent years, notably with regard to states of absorption and trance in both clinical and musical contexts. In an attempt to grasp the diversity and dynamics of subjective experience, recent psycho-phenomenological investigations generally adopt a network approach to the identification, quantification and description of states of consciousness, which are seen as unique, recognizable and temporarily stable combinations of key phenomenal dimensions (e.g., self-awareness, attention, mental imagery, intentionality) (Gabrielsson & Wik, 2003). Moreover, rather than the aggregation of experiential attributes, a state of consciousness is conceived as the emergent consequence of multivariate relationships between dimensions, with its attributes varying in importance and the overall configuration of the state possibly differing across contexts (Vroegh, 2021). Thus, finding its way from neuroscience to psycho-phenomenology, network analysis is a promising method for explaining the phenomenological properties of complex consciousness.
Critically evaluating theories of consciousness through adversarial collaborationWhile IIT and GNWT generally privilege the explanation of different aspects of consciousness – those being global consciousness and perceptual consciousness, respectively – these theories still overlap extensively in their explananda, namely in terms of the neural basis of perceptual consciousness, often maintaining incompatible predictions. Having evolved in parallel, these theories independently accrued evidence using distinct experimental design choices, raising concerns of confirmation bias.
Aiming at accelerating progress in consciousness research, Melloni et al. (2023) notably devised a large-scale, open science, adversarial collaboration between IIT, GNWT and theory-neutral researchers, which jointly developed an experimental design to test some divergent predictions of these two theories. This included preregistering the experimental method, predictions, expected outcomes, and their eventual interpretation.
The first diverging prediction concerns the brain areas in which conscious content is decoded. While IIT attribute this function to posterior cortical areas, GNWT privileges the prefrontal cortex. The second pertains the maintenance of conscious content over time. If, on the one hand, IIT predicted that this content is continuously maintained by neural activity in the temporo-parietaloccipital ‘hot zone’ as long as conscious experience persists, on the other hand, GNWT predicted that an ignition in the prefrontal cortex marks conscious content onset and offset, while in between information of this conscious content is maintained “silently”. The last divergent prediction concerns the inter-areal communication between cortical regions during conscious perception. IIT predicted short-range connectivity within the posterior cortex (i.e. between high-level and low-level sensory areas), while GNWT privileged long-range connectivity between high-level category-selective areas and the prefrontal cortex. To test these predictions, a visual perception task was employed in a large sample (n = 256), involving the visualization of suprathreshold stimuli for variable durations while brain activity was recorded using functional magnetic resonance imaging, magnetoencephalography, and intracranial electroencephalography.
Regarding the first prediction (decoding of conscious content), results corroborated IIT's prediction. Indeed, posterior cortical areas displayed maximal decoding of category and orientation of percepts, suggesting that these areas are sufficient for visual conscious experience. At the same time, the prefrontal cortex showed a consistent representation of category, but not of identity (with representation of orientation being evident only in MEG), casting doubts as to whether the prefrontal cortex is involved in broadcasting all aspects of conscious content, as GNWT predicts (Mashour et al., 2020), or only a subset. As for the second prediction (maintenance of conscious content over time), results partly corroborated IIT's position, with most information concerning visual experience being continuously maintained in posterior areas except for representation of orientation, a nonetheless fundamental property of conscious visual experience in IIT's framework (Haun & Tononi, 2019) that warrants further investigation. GNWT's prediction, on the other hand, is challenged by the lack of ignition at stimulus offset, a salient update on conscious experience that remains unaccounted for within GNWT's framework. Finally, regarding the third prediction (inter-areal synchrony), results showed a lack of sustained synchronization within posterior cortex, strongly refuting IIT's prediction. In addition, a transient increase in long-distance functional connectivity between the prefrontal cortex and face and object-selective posterior areas was observed, corroborating GNWT's stance.
In sum, this initiative shows how a multimodal adversarial collaboration moderated by theory-neutral researchers can boost progress in consciousness research by fostering more creative experimental design choices, potentially leading, as illustrated above, to surprising results that warrant extensive reformulation of theoretical frameworks.
Concluding remarksWe have seen that an embodied central nervous system capable of processing and integrating information is a necessary condition for “global consciousness”. However, global consciousness is a necessary but not a sufficient condition for “perceptual consciousness”. Being conscious of “something” requires processing of specific perceptual signals, both external and internal (external stimuli and affering internal stimuli), in re-entrant and feedback circuits within and among modular and multi-modular brain regions, that render the information available to the whole organism. Finally, “self-consciousness” requires that the brain is capable of locating information inside the body and within the mind. Information integration, information availability and information location are three important prerequisites for any system to be conscious at all, being conscious of something, and being conscious of itself (Fig. 5).
Here, we have delineated the road ahead of us for re-establishing psychology as a science of consciousness, where the study of complex consciousness experiences – whether on the brain level or from a psycho-phenomenological perspective - could play a key role in further discerning the mechanisms associated with global consciousness, the relationship between perceptual and self-consciousness, and the interface among distinct self-consciousness domains.