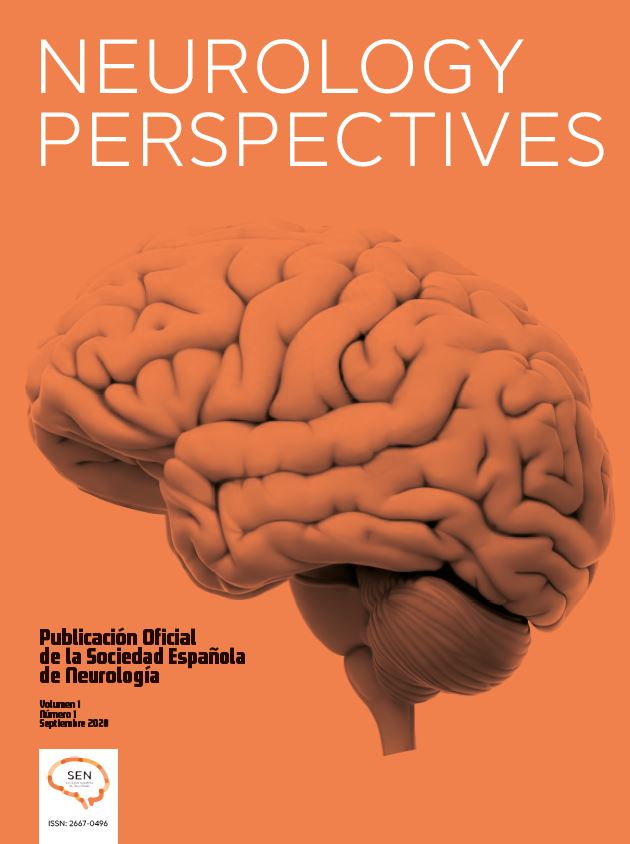
Biomaterial and stem cell -based treatment strategies for diseases of the central nervous system
Más datosNeisseria meningitidis is a bacterium that causes meningitis, a potentially fatal and irreversible disease. Current management of the infection is inefficient treatment based on antibiotics, and is influenced by several factors including the time taken to eliminate the causal agent, prevention of recurrent infections, and the difficulty of reaching the infection site and maintaining proper concentrations of the drug after crossing the blood–brain barrier (BBB); therefore, it is essential to develop new pharmacological alternatives capable of crossing the BBB and avoiding antibiotic resistance. To date, little research has been conducted in the development of organic and inorganic nanostructures to facilitate the delivery of antibiotics and other drugs to the target organ across the BBB, and for the diagnóstico of meningitis.
DevelopmentWe conducted a literature search for nanotechnologies developed for use as direct treatment alternatives against N. meningitidis and to diagnose meningitis caused by this pathogen. We searched various databases and used search engines including NCBI-PubMed and Scopus. The information presented offers a critical overview of the use of nanostructures for antibacterial treatment and diagnóstico.
ConclusionsWe identified a limited number of nanotechnological advances for the diagnóstico and treatment of N. meningitidis infection.
Neisseria meningitidis is a bacterium that clusters in diplococci with a “kidney” or “coffee-bean” shape; its size varies from 0.6 to 1 μm. It is a bacterium of significant medical relevance, as it is the main causal agent of meningitis. N. meningitidis is harboured in the nasopharynx in healthy individuals; nonetheless, in adults, it is considered the second leading cause of meningitis and can progress to sepsis or bronchopneumonia in patients with lung disease.1 Meningococcal infection can be catastrophic; if treatment is not started early, it may cause neurological disorders, and eventually death.2
According to data from the World Health Organization, the most frequent symptoms after meningococcal infection include photosensitivity, neck rigidity, headache, high fever, vomiting, and confusion, although the infection may also cause hearing loss, learning difficulties, and brain damage in 10%–20% of survivors.3 Clinical manifestations can vary according to patient age, with younger patients presenting subtler and less specific symptoms.4 However, 5%–10% of the population are asymptomatic carriers of meningococcal bacteria, outside of epidemic periods.5
N. meningitidisN. meningitidis belongs to the family Neisseriaceae; the bacterium is Gram-negative, non-sporulating, and immobile, and possesses a polysaccharide capsule (Fig. 1). It is classified into 13 serogroups, according to the type of polysaccharide capsule: serotypes A, B, C, D, H, I, K, L, W135, X, Y, Z, and 29E. Serogroups A, B, C, Y, and W135 account for the majority of infections.1
N. meningitidis is also associated with other virulence factors related to the mechanism of infection, including type IV pili, porins, and class 5 proteins (Opa and Opc), among others (Table 1).6
Virulence factor | Function |
---|---|
Type IV pili | Promote adhesion to epithelial and endothelial cells |
Polysaccharide capsule | Protects the bacterium against the host inflammatory response, such as complement activation and phagocytosis-induced cell death; increases resistance against antimicrobial peptides |
PorA | Surface adhesin that helps the bacterium to bind to the cerebral vascular endothelium |
PorB | External membrane porin that works as an entry or excretion channel for antimicrobial peptides |
Class 5 proteins (Opa and Opc) | In non-encapsulated bacteria, they mediate the interaction between the bacterium and eukaryotic cells.In encapsulated bacteria, they facilitate interaction with epithelial, endothelial, and polymorphonuclear cells.Opa proteins bind to CD66 receptors and mediate adhesion and cell invasion. |
Lipid A | Lipopolysaccharide that confers resistance against antimicrobial peptides |
Mtr efflux pumps | Reduce susceptibility to polymyxin B, LL-37,⁎ and antimicrobial peptide PG |
Factor H binding protein (fHbp) | External membrane lipoprotein enabling the bacterium to evade the innate immune system |
IgA1 protease | Assists in colonisation of the mucosa and bacterial survival |
Under the microscope, N. meningitidis is observed in diplococci (also known as meningococci), whose growth requires demanding nutritional conditions: a humid atmosphere on chocolate agar or blood agar media, temperature between 35 °C and 37 °C, and 5% CO2; colonies are small (1–5 mm) with convex morphology, elevated, mucoid, opaque or transparent, and do not present haemolysis. Complementary biochemical findings for identification and differentiation of the pathogen are positivity for oxidase and catalase, and acid production due to the oxidation of glucose and maltose.1
EpidemiologyDisease due to meningococcal infection is a global problem that is endemic in many countries. The main risk groups are children younger than 5 years of age, patients with complement factor deficiencies, and immunosuppressed patients, as well as smokers, employees of microbiology laboratories, and tourists visiting high-risk areas.1,7 The groups most frequently affected are infants younger than one year old and young people aged 15–19 years; epidemics also occur in the so-called meningitis belt in Sub-Saharan Africa.2 Serotypes A, C, and W135 are the predominant forms in Africa; serotypes A, B, and C in Asia; serotypes B and C in Europe; serotypes B, C, and Y in North America; serotypes B and C in South America and Australia; and serotype B in New Zealand.2 Serotype W135 recently overtook serotype B, which was previously responsible for over 60% of cases: in 2013, the former serotype accounted for 62.5% of cases.7,8
Pathophysiology of bacterial meningitisPerson-to-person transmission of N. meningitidis mainly occurs via small droplets from the throat or respiratory secretions; the bacteria crosses the non-ciliated epithelial cells of the upper respiratory mucosa via adhesins, pili, and opacity-associated proteins (Opa and Opc) and infects phagocytic cells, which it uses as a “Trojan horse,” ceasing production of the capsule and promoting bacterial survival.2,6–8,12 After entering the blood, the pathogen can multiply rapidly, spreading throughout the body until it reaches the brain.2,5 Nonetheless, it is considered a commensal pathogen of the nasopharyngeal epithelium; the factors explaining the appearance of meningococcal disease are unknown.7,8
As mentioned previously, after entering the blood, the pathogen may reach the brain, where it induces the release of components of the bacterial cell wall or membrane, stimulating astrocytes, microglial cells, and vascular endothelial cells to generate proinflammatory cytokines. This results in a lesion to the vascular endothelium, increasing the permeability of the blood–brain barrier (BBB); the subsequent massive generation of leukocytic infiltrate in the subarachnoid space and induction of brain oedema leads to intracranial hypertension and reduced cerebral blood flow.8,9
These alterations cause hypoxia, increased anaerobic metabolism (with lactate production), and decreased glucose consumption. If this process continues, endothelial damage triggers vascular thrombosis, resulting in a further reduction in local cerebral blood flow, with areas of ischaemia and cerebral infarction.2
Diagnosis, treatment, and preventionDiagnosis begins with a physical examination, and subsequently microbiological analysis with a blood agar culture of cerebrospinal fluid (CSF) obtained by lumbar puncture, and sometimes also a culture of nasopharyngeal exudate.7 If results from the CSF culture are positive, rapid detection of bacterial capsular antigens is useful.7 Aetiological diagnóstico may be affected by false negative results due to onset of antibiotic treatment prior to blood and CSF sampling; however, the cytochemical characteristics of the CSF are not affected.4 Molecular diagnóstico is conducted by detection of meningococcal genetic material with polymerase chain reaction (PCR) testing, a highly sensitive and specific technique.1
The antibiotics of choice for treating N. meningitidis infection are penicillin G and ampicillin. Patients with allergies to these compounds are treated with such third-generation cephalosporins as ceftriaxone or cefotaxime. Other antibiotics used to treat this infection include chloramphenicol, fluoroquinolones, and meropenem.4,7,10 Another recommended treatment is immunomodulatory therapy with dexamethasone followed by antibiotic treatment; this approach reduces rates of mortality in adult patients and such sequelae as severe hearing loss in children. However, dexamethasone reduces the penetration of vancomycin. Finally, a second CSF analysis should be conducted 24–48 h after the course of treatment is completed to verify elimination of the microorganism.4,10
Regarding prevention, several types of vaccines have been available for over 30 years for serotypes A, C, Y, and W135. A vaccine was recently developed against serotype B,3 and presents a structural similarity with glycans on human neuronal-cell adhesion molecules, as N. meningitidis presents surface modulation and point mutations.2,8
To prevent secondary cases, antibiotic prophylaxis is administered to all individuals who were in contact with the patient for longer than 8 h or who due to close proximity with the patient may have been exposed to secretions, with a transmission window from one week before symptom onset to 24 h after onset of antibiotic therapy.7
Nanomaterials in N. meningitidis meningitisOne of the main issues in treating central nervous system (CNS) diseases is drug administration. The most viable method for delivering drugs to the CNS is the intranasal route, which is permeable, richly vascularised, and presents a large area for absorption, enabling drugs to reach the systemic circulation, bypassing gastric degradation and hepatic metabolism. This method includes 3 pathways: in the first, the drug is absorbed, reaches the systemic circulation, and crosses the BBB; in the second, it crosses the olfactory epithelium to the olfactory bulb and subsequently reaches the CSF; and in the third, it bypasses the BBB and is transported directly to the CNS via the trigeminal nerve.11 However, macromolecular drugs cannot cross the tight junctions of the BBB, limiting their bioavailability in the brain.12
Nanotechnology is a discipline that enables the creation of structures at the nanometre scale and promises to resolve a series of issues in the diagnosis and treatment of many neurological diseases, including meningitis. Several types of nanomaterials have been studied for the treatment and diagnosis of meningitis; most are organic, in the form of nanospheres or nanocapsules (Fig. 2A).11 The properties of these nanomaterials allow for the administration of drugs with better chances of crossing the complex physiology of the BBB (Fig. 2B).12,13
Nanomaterials used in Neisseria meningitidis infection. A) Some of the nanoparticles used in the development of nanosensors and nanocarriers for the diagnóstico and treatment of N. meningitidis infection, respectively. B) Diagram of the blood–brain barrier, which the bacterium must cross in order to cause meningitis, and which nanomaterials must cross in order to treat the disease.
Nanostructures are also highly precise and specific to receptors for the purpose of active targeting; this makes them ideal delivery systems for penetrating the BBB. Furthermore, nanomaterials with biomimetic functions have been developed that are able to efficiently cross the BBB, where they are engulfed by pathogens.12
The following types of nanostructures have been used for diagnostic and therapeutic purposes:
Graphene oxideGraphene, a 2-dimensional structure made up of carbon atoms, is obtained from graphite. The quality, quantity, size, and structure of graphene layers vary according to the intended application. Chemical exfoliation of graphite enables the production of graphene derivatives, such as graphene oxide (GO). This material presents excellent physicochemical characteristics that are desirable in medical diagnóstico, such as sensitivity and safety in the design of nanosensors.
For detecting N. meningitidis, it has been suggested that GO may be used to create a nanosensor comprising a single-strand DNA (ssDNA) probe for the pathogen supported by graphene oxide with a poly(methyl methacrylate) microfluidic chip for efficient, precise detection of the microorganism.4
Silicon dioxide nanoparticlesSilicon-based nanomaterials, such as silicon dioxide nanoparticles, have been used for the diagnóstico and treatment of a range of diseases, with excellent results. The unique surface of the material makes it attractive as a carrier or for conjugating multiple organic molecules, such as nucleic acids and proteins. Phaneuf et al.10 reported promising results with diagnóstico using the RNA-guided CRISPR-Cas9 nuclease system coupled with functionalised silica microparticles.
Zinc oxide nanoparticlesZinc oxide (ZnO) presents several properties that make it interesting from a diagnostic perspective, as it can be used to produce sensors due to its suitable physicochemical properties: electronic transference, catalytic efficiency, strong chemical stability, high isoelectric point, and biocompatibility; it also presents such biological properties as antiviral, antibacterial, and anticancer activity. Regarding its diagnostic use, one study reports promising results with a ZnO- and multiwall carbon nanotube–based nanocomposite matrix on an indium tin oxide–coated glass platform for the identification of N. meningitidis.5
Lipidic nanocarriersLipidic nanocarriers are liposomal nanostructures with a phospholipidic composition, making them biodegradable, non-immunogenic, non-toxic, and capable of penetrating membranes. Due to their unique composition, nanoliposomes can be loaded with both hydrophobic and hydrophilic drugs. Specifically, some treatments for meningitis have been developed that use lipid-based nanocarriers; one was reported in 2015 by Stalmans et al.,14 who found that nanoliposomes loaded with phosphoethanolamine are able to penetrate the membranes of Gram-negative bacteria with efficient bactericidal activity. A recent study analysed the combined effect of the antibiotics levofloxacin and doxycycline loaded on lipidic nanocarriers and delivered to the brain via the intranasal route to treat meningitis, with convincing results.15
Carbon nanotubesLike fullerenes, diamonds, and graphite, nanotubes are allotropes of carbon. Their structure is achieved by rolling a sheet of graphene, with different diameters and geometries. Depending on the number of graphene layers, carbon nanotubes may be single-wall (SWCNT) or multi-wall (MWCNT). This is an optimal material whose surface electrical properties make it useful for fabricating super-miniaturised chemical and biological sensors. Recent studies by Dash et al.16,17 report the use of MWCNTs to develop sensors for detecting N. meningitidis with a view to reducing the incidence of these infections. The same authors used a 1-octadecanethiol-carboxylated MWCNT composite with an ssDNA probe for detecting N. meningitidis; this molecule functions as a genosensor for the rmpM gene of the pathogen in CSF samples.
Polymeric nanostructuresPolymer-based nanostructures are important molecules and are commonly used as sensors. Two candidate materials for diagnostic purposes are artificial polymers (polymersomes) and planar polymer membranes due to their tunability and nanoporosity, respectively.18 This type of polymeric nanostructure has been used to develop a polymer/paper hybrid microfluidic biochip with loop-mediated isothermal amplification (LAMP) for the detection of N. meningitidis; the technique showed high sensitivity and specificity.4
Silver nanoparticlesSilver is known to have been used as an antimicrobial agent since antiquity, mainly in disinfecting water and treating chronic wounds. The introduction of antibiotics in 1940 led to a reduction in its use; however, due to bacterial resistance, recent years have seen a resurgence in the use of silver in the form of nanoparticles.19
Silver nanoparticles can now be generated by extracellular synthesis with widely studied bacteria such as Escherichia coli and Bacillus subtilis and the yeast Saccharomyces cerevisiae. These particles have been shown to be effective against N. meningitidis in vitro, leading to the evaluation of their use as an alternative to antibiotic treatment.9,20
ConclusionEarly diagnóstico and treatment of N. meningitidis infection is fundamental to ensuring good health outcomes in these patients. Due to the difficulty of reaching the infection site, it is essential to find or develop new alternatives for delivering drugs across the BBB. In this respect, the development of new nanotechnologies that function as antimicrobial agents or diagnostic nanosensors makes these materials a promising line of research in diseases of the CNS, precisely because of the physicochemical properties that result from their small size. The most valuable properties of the nanomaterials used in the treatment and diagnóstico of N. meningitidis infection are their ability to function both superficially and internally, their capacity to cross cell membranes, their high surface area–to-volume ratio, and the increased release of drugs. Much research is yet to be conducted, as few studies have addressed the use of nanostructures with antimicrobial potential against N. meningitidis, and no study has analysed the mechanisms underlying their bactericidal effect.