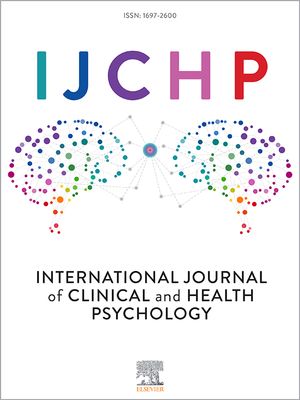
Editado por: Andre R Brunoni, Marie-Anne Vanderhasselt, Leigh Chavert
Más datosEvidence indicates beneficial effects of aerobic exercise on motor learning performance, which might be caused by the impact of aerobic exercise on cortical excitability. It is thus suggested that physiological effects of aerobic exercise on cortical excitability determine the effects of aerobic exercise on motor learning. Nevertheless, respective results usually come from independent studies, and a prove of the causal relationship between neurophysiological and motor learning effects is still missing. This study aims to explore the impact of a single bout of aerobic exercise on brain physiology and motor learning, and the association between these phenomena in humans
MethodThe study was conducted in a cross-over design. In twenty healthy subjects, cortical excitability and motor learning were assessed before and after a single bout of aerobic exercise or a control intervention
ResultsThe results show that aerobic exercise improved motor sequence learning and enhanced cortical excitability in humans. Furthermore, a correlation between the exercise-dependent alteration of cortical excitability (short intracortical inhibition, which is determined primarily by the GABAergic system) and improvement of motor learning has been found
DiscussionThe study found motor learning performance-improving effects of aerobic exercise, and these results might be explained by an exercised-caused alteration of cortical excitability, especially a reduction of GABA activity.
Motor learning is crucial in human daily life, both throughout the lifespan as well as for the recovery from a variety of neurological diseases (Krakauer, 2006). Converging evidence suggests that a single bout of aerobic exercise is sufficient to lead to immediate improvements of motor learning in healthy subjects as well as in clinical populations such as stroke (Imboden et al., 2019; Quaney et al., 2009; Snow et al., 2016). The foundation of these effects might be the impact of aerobic exercise on neuroplasticity and cortical excitability (Arcangelo, Triossi, Buglione, Melchiorri, & Tancredi, 2017; Cabral et al., 2019). Animal studies suggest that neuronal excitability is enhanced by exercise via suppressing gamma-aminobutyric acid (GABA)-dependent inhibition and facilitating the activation of glutamatergic N-methyl-D-aspartate (NMDA) receptors (Arcangelo et al., 2017; Yu, Li, Wang, & Li, 2013). Recently, transcranial magnetic stimulation (TMS) paradigms have been applied to evaluate the effects of aerobic exercise on cortical excitability in the motor cortex in humans (Morris et al., 2019; Smith, Goldsworthy, Garside, Wood, & Ridding, 2014). Corticospinal excitability can be assessed by active and resting motor thresholds (MTs) and the input-output curve (I-O curve) (Abbruzzese and Trompetto, 2002a; Chen, 2000b). MTs reflect neuronal membrane excitability and depend primarily on ion channel activity, because they are increased by voltage-gated sodium channel blockers, but not affected by drugs modulating GABAergic or glutamatergic transmission (Ziemann, Chen, Cohen, & Hallet, 1998; Ziemann, Loennecker, Steinhoff, & Paulus, 1996). The input-output curve (I-O curve) serves as an index of excitability of larger neuronal populations compared to MTs (Abbruzzese and Trompetto, 2002b; Chen, 2000a). The I-O curve depends on neuronal membrane excitability, as its slope is decreased by sodium and calcium channel blockers. Furthermore, synaptic mechanisms are involved as it is modulated by drugs affecting the GABAergic and glutamatergic system, especially at higher stimulation intensity (Broojerdi, Battaglia, Meullenbacher, & Cohen, 1999; Lazzoro et al., 2003; Paulus et al., 2008). Short-interval intracortical inhibition (SICI) and facilitation (ICF) can be explored by paired-pulse TMS. SICI is mainly regulated by GABAA, but also glutamate receptors and based on the induction of inhibitory postsynaptic potentials (Liepert, Schwenkreis, Tagenhoff, & Malin, 1997). ICF is thought to control activity of primarily the glutamatergic system (Ziemann, 2004). In previous studies, aerobic exercise enhanced ICF and decreased intracortical inhibition but showed no effect on MTs (McDonnell, Buckley, Opie, Ridding, & Semmier, 2013; Smith et al., 2014). These findings confirm that aerobic exercise affects human brain excitability.
Previous studies show a relevant, and mechanistically related impact of aerobic exercise on motor learning and cortical excitability (Morris et al., 2019; Quaney et al., 2009; Smith et al., 2014; Snow et al., 2016). It is thus suggested that physiological effects of aerobic exercise on cortical excitability determine the impact of aerobic exercise on motor learning processes. Nevertheless, respective results come from independent studies, and the causal relationship between neurophysiological and motor learning effects has not been experimentally investigated directly so far. In the present study, we conducted neurophysiological assessments and explored motor learning performance in identical participants to explore respective associations directly. The study thus aims to explore the impact of aerobic exercise on brain physiology and motor learning, and the association between these phenomena in humans. We hypothesized that a single bout of aerobic exercise improves motor learning and modulates cortical excitability. Furthermore, we expected an association between motor learning and neurophysiological data.
Methods & materialSubjectsTwenty healthy right-handed non-smokers (10 females) aged 26.4±1.01 years (mean ± SD) were recruited. None of them had a history of neurological and psychiatric diseases, pregnancy, or metallic head implants, nor did they take any medication during the study period. Written informed consent was obtained from all participants before inclusion. The investigation was approved by the ethics committee, and conforms to the Declaration of Helsinki.
Aerobic exercise interventionParticipants were required to pedal a stationary exercise bike. Before exercise, baseline heart rate (HR) was obtained. During exercise, HR was monitored by a wrist-mounted pulse rate sensor. Participants were instructed to perform at 61-74% of their age-predicted maximal heart rate HR (i.e. 220-age). The exercise consisted of: (1) 5 min warm-up exercise, (2) 20 min exercise at target HR, and (3) 5 min cool down exercise. Throughout the exercise period, HR was monitored continuously. In this study, not VO2 peak, but HR was used to determine individual exercise intensity, which is in line with foregoing studies (Balter A et al., 2018; Singh A et al., 2014). The chosen exercise intensity level of 61-74% HR max is in further accordance with previous studies exploring the impact of aerobic exercise on cortical excitability in healthy adults (Baltar, Nogueria, Marques, Carneiro, & Monte-Silva, 2018; Lulic, El-Sayes, Fassett, & Nelson, 2017; Singh, Neva, & Staines, 2014). The exercise duration and protocol characteristics (5 min warm up, 20 min exercise, and 5 min cool down) was likewise chosen in accordance with a previous study exploring the impact of aerobic exercise on cognitive performance in healthy adults (Chang, Chi, Wang, Chu, & Zhou, 2014). In the control condition, participants were resting for 30 min. During this time, participants could interact with study staff or read, but were not allowed to conduct whole-body movements. HR was monitored during the rest condition.
Motor learning task: serial reaction time task (SRTT)
Participants were seated in front of a computer screen at eye level and a response pad placed on a table with four buttons numbered 1–4. They were instructed to push each button with a different finger of the right hand (index finger for button 1, middle finger for button 2, ring finger for button 3, and little finger for button 4). In each trial, an asterisk appeared in one of 4 positions that were horizontally spaced on a computer screen and permanently marked by dots. Participants were instructed to press the key corresponding to the position of the asterisks as fast and correct as possible. After a button was pushed, the go signal (asterisk) disappeared. The next go signal was displayed 500ms after the participant pushed the button, without giving information if the answer was correct or not. A test session consisted of 8 blocks of 120 trials each. In blocks 1 and 6, the sequence of asterisks followed a pseudo-random order. Asterisks were presented equally frequently in each position and never in the same position in two subsequent trials. In blocks 2–5, and 7 and 8, the same 12-trial sequence of asterisk positions was repeated for 10 times. Participants were not told about the repeating sequence. Parallel task versions were applied, and the task versions were randomized to intervention conditions and intervention order (Grundey et al., 2015; Nitsche et al., 2003).
Motor cortical excitabilityMotor evoked potentials (MEPs) were induced in the right abductor digiti minimi muscle (ADM) by single-pulse TMS over the left primary motor cortex. TMS was conducted by a Magstim 200 magnetic stimulator (Magstim Company, Whiteland, Dyfed, United Kingdom) with a figure-of-eight magnetic coil (diameter of one winding=70mm; peak magnetic field=2.2 T). For the paired-pulse TMS protocols, the coil was connected to two Magstim 200 stimulators via a bistim module. The coil was held tangentially to the skull, with the handle pointing backwards and laterally at 45° from midline. The individual optimal coil position was defined as the site where TMS with medium intensity resulted consistently in the largest MEP of the target muscle. Surface electromyography (EMG) was recorded from the right ADM by use of Ag-AgCl electrodes in a belly tendon montage. The signals were amplified, and band-pass filtered (2Hz to 2 kHz; sampling rate, 5 kHz). Signals were digitized with a power 1401 data acquisition interface (Cambridge Electronic Design, Cambridge, United Kingdom) and stored for offline analysis. The resting motor threshold (RMT) was defined as the minimum TMS intensity which elicited a peak-to-peak MEP of 50-100 μV in the relaxed muscle in at least three of six consecutive trials. The active motor threshold (AMT) was the minimum intensity eliciting a MEP response of ∼200-300 μV during moderate spontaneous background muscle activity (∼15% of the maximum muscle strength) in at least three of six consecutive trials. The I-O curve was determined using TMS intensities of 100, 110, 130, and 150% RMT (15 stimuli per intensity block, with the order of the blocks randomized), in accordance with previous studies (Antal, Terney, Kühnl, & Paulus, 2010; Grundey et al., 2015; Ragert, Camus, Vandermeeren, Dimyan, & Cohen, 2009). Short-latency intracortical inhibition and facilitation were measured by a TMS paired-pulse protocol including ISIs of 2, 3, 5, 10, and 15 ms. The first three ISIs represent inhibitory, and the last two ISIs facilitatory effects, which reflect excitability of inhibitory and excitatory interneurons, respectively. In this protocol, the subthreshold conditioning stimulus (intensity determined as 70% of AMT) precedes the test stimulus. The test pulse was adjusted to achieve a baseline MEP of ∼1mV and readjusted during the respective stimulation protocols, if needed, to compensate for effects of global excitability changes on test pulse amplitude. The pairs of stimuli were organized in 15 blocks, and each block included one single pulse, and one of all double pulse conditions in pseudo-randomized order. Regarding the intensity of the conditioning stimulus, we applied 70 % of AMT (Ziemann, Rothwell, & MC, 1996), to avoid ceiling effects, which might limit the observation of intervention-based excitability alterations..
Experimental courseThe study was divided into two parts, each with two experimental sessions (Figure 1). Within each part, sessions were carried out in counterbalanced order and separated by at least one week. All volunteers completed both parts of the study (four sessions). A questionnaire-based interview (asking whether the participants felt fatigue, headache, or dizziness) was applied after aerobic exercise intervention. The first part (two sessions) of the experiment explored effects of aerobic exercise on motor leaning. The parallel versions of SRTT were assessed before and after the intervention (AE: aerobic exercise or Con: control condition). The second part (two sessions) of the experiment explored the effects of aerobic exercise on corticospinal and intracortical excitability. Subjects were seated in a comfortable chair with head and arm rests. The hotspot of the right ADM was determined over the left primary motor cortex, and 20 MEPs were recorded with the TMS intensity which elicited on average MEP of 1mV amplitude (SI1mV). Afterwards, RMT and AMT were determined using standard procedures. After measuring AMT, a 15 min break followed to avoid a possible effect of muscle contraction on the next measurements. After this break, the following parameters were recorded in randomized order as baseline measures: I-O curve and SICI-ICF. All of the above-mentioned parameters were measured before and after intervention.
Experimental course of the study. The study was divided into two parts. The first part (two sessions) of the experiment explored effects of aerobic exercise on motor leaning. Parallel versions of the SRTT were assessed before and after the intervention (AE: aerobic exercise or Con: control condition). The second part (two sessions) of the experiment explored the effects of aerobic exercise on cortical excitability via TMS measurements.
For the SRTT, in each trial, response time (RT) was recorded from the appearance of the go signal until the first button was pushed by the subject. For each block of trials of a given experimental session, mean RT was calculated for each subject separately. Incorrect responses, response times of less than 200 ms or more than 3000 ms, and those that differed more than 3 standard deviations from the individual mean response time were discarded. Mean RT were standardized to block 1 for each subject in each intervention condition separately, to control for initial RT differences. Furthermore, the standard deviation of response times for each subject in every block was calculated as an index of variability of response times. Error rate (ER) was calculated to assess the number of incorrect responses for each block and each subject in each session. Statistical analyses were performed for absolute and standardized values of RT, ER, and variability of RT via repeated measures analyses of variance (ANOVA) (level of significance 0.05), and the within-subject factors intervention condition (AE and Con), and time course (pre-and post-intervention). The Mauchly test was performed to test for sphericity and the Greenhouse-Geisser correction were applied when necessary, for these, and the following ANOVAs. Dependent on significant results of the ANOVAs, RT, ER, and variability differences between the respective intervention conditions were compared by two-tailed Student's t-tests for each block between intervention conditions, and between blocks within an intervention condition. Since RT differences between block 5 and 6 are thought to represent an exclusive measure of implicit learning, interactive Student's t tests were conducted to compare differences of respective RTs between the intervention conditions (blocks 5, and 6 of the SRTT).
With regard to the SI1mV, RMT, and AMT, the individual means of the TMS intensity at SI1mV, RMT, and AMT were calculated for the before and after intervention separately. Repeated-measures ANOVAs were performed for the above-mentioned data using the RMT, AMT, and SI1mV value as the dependent variable, and intervention condition (AE and Con) and time point (pre- and post-intervention) as within-subject factors. For the I-O curve, the individual means of MEP amplitudes were calculated for all subjects. Repeated-measures ANOVAs were performed for the above-mentioned data using MEP amplitudes as the dependent variable, and intervention condition (AE and Con), time point (pre- and post-intervention), and TMS intensity as within-subject factors. Regarding SICI-ICF, the mean values were normalized to the respective single-pulse condition. First, intra-individual means were calculated for each condition. To determine significance, repeated-measures ANOVAs were performed (ISIs, intervention conditions, time point as within-subject factors and MEP amplitude as the dependent variable). In case of significant results of the ANOVA, exploratory post hoc comparisons were performed using Student's t tests (2-tailed, P<.05). To explore a priori differences between conditions, a 2-factorial model ANOVA were conducted to compare the baseline MEP amplitudes between intervention conditions (AE and Con).
Simple bivariate correlations (Pearson's r coefficient) were conducted to explore the relationships between the change of motor learning (performance block 5/6) and the change of neurophysiological parameters (SI1mV, RMT, AMT, I-O curve: means of different intensities, SICI: means of different ISI, and ICF: means of different ISI) under aerobic exercise intervention (pre_AE vs. post_AE).
ResultsAll subjects tolerated the exercise protocols well. According to the questionnaire-based interview (fatigue, headache and dizziness after exercise), none of the participants reported any side effects after aerobic exercise intervention.
SRTTAs displayed in Table 1, for absolute RT, the repeated measures ANOVA revealed significant main effects of the factors block (F(7)=11.344; P=0.001), condition (F(1)= 10.217; P=0.002), time (F(1)=5.613; P=0.003), and significant condition x block (F(7)=54.212; P<0.001), block x time (F(7)=11.43; P=0.002), condition x time (F(7)=5.523; P=0.039), and block x condition x time (F(7)= 8.32; P=0.001) interactions emerged. For standardized RT, significant main effects of the factors block (F(7)=51.549; P<0.001), condition (F(1)= 52.676; P<0.001), time (F(1)=50.662; P<0.001), and significant condition x block (F(7)=48.32; P<0.001), block x time (F(7)=51.323; P<0.001), condition x time (F(1)=48.92; P<0.001), block x condition x time (F(7)=44.89; P<0.001) interactions emerged. For absolute RT, the main effect of block was caused by shorter RT in the sequence blocks compared to the random blocks in all conditions (Figure 2), with the exception of the random block 6, which did not contain the learned sequence, in all exercise conditions. The main effects of the factors condition and time were caused by shorter RT for the AE_post as compared to other conditions, and shorter RTs in all conditions in the later, as compared to the earlier blocks (Figure 2A). Similar results were obtained for standardized RT. Standardized RTs were significantly smaller under AE_post as compared to the other conditions (Figure 2B). With respect to the significant interactions, as revealed by the post hoc t-tests, the difference of absolute and standardized RT between block 6 and 5 (RT6-RT5) was significantly larger in the AE_post condition than in other conditions, reflecting improved learning in the aerobic exercise condition. For ER and variability, the respective repeated measures ANOVAs showed no significant main effects of condition, block or the respective interactions (all P>0.05, Table 1).
Repeated-measures ANOVA results for motor learning (SRTT).
Abbreviations: RT=reaction time *Significant results at p<0.05, d.f.: degrees of freedom.
SRTT performance (reaction time). Depicted are the (A) mean absolute reaction time (ms) and (B) standardized reaction time for each intervention condition (blocks 1-8). In blocks 1 and 6, random stimuli, and in the remaining blocks, the sequence was presented. The results show that participants learned in all conditions (aerobic exercise (AE) and control (Con)). In addition, reaction time was generally significantly shorter in the AE_post condition as compared to Con_post (block 3, 4, 5, 7 and 8). For both, A and B, the reaction time difference between block 5 and 6, which is a pure index of motor sequence learning, was larger for the AE_post, as compared to Con_post, indicating improved learning under aerobic exercise. Filled symbols indicate significant reaction time differences within respective intervention conditions relative to block 1 (2-tailed t tests, paired samples, P < 0.05). The asterisks indicate significant differences between the AE_post vs Con_post conditions for a single block (2-tailed t tests, paired samples, P < 0.05). The floating circle symbols indicate significant differences between the AE_post vs AE_pre conditions within a block (2-tailed t-tests, paired samples, P <0.05). The hash sign indicates a significant difference of the RT difference between block 5 and 6 with respect to the AE_post vs Con_post conditions (2-tailed, t-test, paired samples, P <0.05). Error bars in this and the following figures represent standard error of the mean (SEM).
For TMS data, there were no significant differences of baseline MEP amplitudes between conditions (all P>0.05). SI1mV, motor thresholds (AMT and RMT), and the slope of the recruitment curve show no significant effects of the main factors condition and time or the respective interactions (p> 0.05) (Table 2). For SICI and ICF, the ANOVA revealed significant main effects of condition (F(1)= 9.212; P<0.001), time (F(1)= 8.589; P=0.001), ISI (F(4)= 4.171; p=0.002), and the condition x time (F(4)= 12.697; p< 0.001), condition x ISI (F(4)= 11.481; p<0.001), Time x ISI (F(4)= 6.232; p=0.001), and condition x time x ISI (F(4)= 5.376; p=0.001) interactions (Table 1). Post hoc Student's t tests (two-tailed, p < 0.05) show that AE enhanced intracortical excitability. Aerobic exercise significantly increased facilitation at ISIs of 10ms, and 15ms, and decreased inhibition at ISIs of 2 and 3 compared to the AE_pre and Con_post conditions (Figure 3).
Repeated-measures ANOVA results for cortical excitability (TMS).
Abbreviations: RMT=resting motor threshold; AMT=active motor threshold; I-O curve=input-output curve; SICI-ICF=short-latency intracortical inhibition and intracortical facilitation. *Significant results at p<0.05, d.f.: degrees of freedom.
Short-latency intracortical inhibition and intracortical facilitation before and after intervention (aerobic exercise and control). Single pulse-standardized double pulse stimulation MEP amplitude ratios ± SEM are depicted for ISIs revealing inhibitory (ISIs of 2, 3, and 5 ms) and facilitatory (ISIs of 10 and 15 ms) effects for different conditions: before aerobic exercise (AE_pre), after aerobic exercise (AE_post), before control (Con_pre), and after control (Con_post). In the AE_ post condition, facilitation for the ISI of 10 and 15 milliseconds were significantly increased, and inhibition for ISIs of 2 and 3 milliseconds were significantly decreased compared other conditions. Filled symbols indicate significant differences between post-intervention vs pre-intervention within respective intervention conditions (2-tailed t-tests, paired samples, P <0.05). The asterisks indicate significant differences between AE_post vs Con_post conditions for respective ISIs (2-tailed t tests, paired samples, P < 0.05). Vertical bars depict SEM.
There was a significant negative correlation between the change in SICI (means of different ISIs) and the change in motor learning RT (r= -0.817, p=0.047) under aerobic exercise conditions (Figure 4). However, no association between the change in ICF and motor learning RT was observed (r=-0.38, p=0.072), nor were there significant correlations between the changes in motor learning RT and SI1mV (r=0.15, p=0.66), RMT (r=-0.1, p=0.78), AMT (r=-0.09, p=0.82), and I-O curve (r=0.29, p=0.44).
DiscussionIn the present study, a single bout of aerobic exercise improved motor sequence learning in healthy adults. In the same subjects, exercise furthermore enhanced cortical excitability. It increased intracortical facilitation, and decreased inhibition. In addition, a negative correlation between SICI and improvement of motor learning performance was shown, whereas no correlation was found between ICF or other neurophysiological parameters and motor learning.
Our findings show that RT was selectively shortened for the motor sequence after aerobic exercise, as compared with the control and pre-exercise conditions. This result is in accordance with previous studies, where a single dose of aerobic exercise improved motor learning of continuous tracking, and motor sequence tasks (Snow et al., 2016; Statton, Encamacion, Celnik, & Bastian, 2015). Moreover, the interactive t-test (comparing sequence block 5 and random block 6) conducted for standardized RT revealed a significant difference of AE_post vs. Con_post and AE_pre vs. AE_post, and thus prove a sequence learning-specific effect of this intervention. Additionally, the RT improvement was specific for sequence learning and did not reflect a general RT improvement. ER and variability were not affected by the intervention. Thus, these effects reflect a clear performance improvement, and not improved RTs on the cost of error enhancement.
Regarding corticospinal excitability, AMT, RMT, as well as the I-O curve did not differ between aerobic exercise and control conditions. This finding is in accordance with those of previous studies (Smith et al., 2014; Yamazaki et al., 2019). We found however enhanced ICF as well as a decreased short latency intracortical inhibition after aerobic exercise. ICF is regulated predominantly by glutamate receptors with some GABAA receptor contribution (Ziemann, Chen, Cohen, & Hallet, 1998a; Ziemann, Loennecker, & paulus, 1995; Ziemann, Loennecker, et al., 1996). SICI is suggested to be primarily controlled by GABAA receptors (Ilic et al., 2002; Paulus et al., 2008; Ziemann et al., 1998b). Our results thus imply that aerobic exercise exerts modulatory effects on both excitatory glutamatergic neurotransmission and GABA-related inhibition. These results are in accordance with those of former in vivo and in vitro studies showing that aerobic exercise suppresses GABAergic inhibition and enhances glutamatergic facilitation (Arcangelo et al., 2017; Yu et al., 2013). Previous evidence has shown that exercise modulates relevantly brain physiology, and that intensity, frequency, and duration of exercise determine its efficacy (Lulic et al., 2017; Moscatelli et al., 2020; Yamazaki, Sato, Yamashiro, et al., 2019). The comparative importance of these parameters has however not been explored systematically. Preliminary studies of our own group and others have shown that a single bout of aerobic exercise increases ICF whereas it decreases SICI (T. Lulic, J. El-Sayes, H. Fassett, & A. Nelson, 2017; McDonnell et al., 2013; Mooney et al., 2016; Singh, Duncan, Neva, & Staines, 2014; Smith et al., 2014; Yamazaki et al., 2019). These findings, however, contrast with one study, in which application of light aerobic exercise did not affect SICI (Morris et al., 2019). Since exercise duration is similar in these studies, a likely explanation for these different results is exercise intensity. Our and previous studies applied moderate intensity exercise whereas light intensity was used in Morris’ study. Additionally, a foregoing study has shown that 20 mins of continuous moderate-, but not low-intensity aerobic exercise was effective at modulating corticospinal excitability (MacDonald et al., 2019), and adds evidence that low intensity exercise might not be sufficient to affect brain physiology relevantly. Furthermore, exercise intensity plays a pivotal role in modulating brain physiology. Balter A et al. (2019) showed that 15 mins moderate-intensity aerobic exercise, but not 30 mins low-intensity aerobic exercise enhance cortical excitability. This suggests that exercise intensity has a higher relevance than exercise duration for modulation of brain physiology. Previous studies showed furthermore that single dose and multiple doses of aerobic exercise modulate brain physiology, but a direct comparison between different repetition rates is still missing, and respective studies should be conducted in future (Lulic et al., 2017; Moscatelli et al., 2020; Yamazaki, Sato, Yamashiro, et al., 2019).
No correlations between the changes of motor learning performance and the changes of SI1mV, RMT, AMT, I-O curve after aerobic exercise intervention were observed. Exercise-mediated decreases of SICI were however correlated with improvements in motor learning performance. No correlation between ICF and motor learning was observed. This finding shows a similar pattern with previous research, which included magnetic resonance spectroscopy (MRS) in sequence learning, and showed that GABA activity is reduced, but glutamate is not altered in the primary motor cortex during learning, and the magnitude of GABA reduction correlates positively with motor learning (Stagg, Bachtiar, & Johansen-Berg, 2011). These results do not imply that the glutamatergic system is not relevant for motor learning, but its effects might be more focal, and therefore escape methods with limited spatial resolution, such as MRS. In general accordance with our results, a foregoing study has described a positive correlation between exercise-increased cortical excitability (MEP amplitude) and non-motor procedural memory consolidation (Ostadan et al., 2016). This finding suggests that aerobic exercise may exert a widespread effect on the brain, and affects learning also with respect to cerebral areas, which show no close connection with the motor system.
The specific mechanisms responsible for the effects of aerobic exercise on motor learning should be further investigated in future studies. Exercise-induced cortical modulation, as outlined above, is a candidate mechanism. Changes in cortical excitability and activity are a necessary precondition for sustained changes in synaptic strength, as present in long-term potentiation (LTP) and long-term depression (LTD) (Daoudal & Debanne, 2003; Malenka & Bear, 2004; Rioult-Pedotti, Friedman, Hess, & Donoghue, 2000). Moreover, LTP is related to learning, depends on glutamatergic mechanisms, and is fostered by GABA reduction. Animal studies have shown that exercise induces glutamatergic and calcium–dependent LTP (Arcangelo et al., 2017). Thus, exercise might have enhanced learning-related plasticity via LTP-enhancement via GABA reduction, and glutamate enhancement. The results of the present study are in line with previous research that a single dose of aerobic exercise increases ICF whereas it decreases SICI. This might create a more favourable environment for task-related long term potentiation-like excitability changes (Singh et al., 2014). The present study adds relevant information that aerobic exercise might modulate neurotransmitter functions, specifically for GABA and glutamate, and that especially the reduction of GABA-related diminution of cortical excitability is closely related to learning.
Some limitations of the present study should be taken into account. This study was conducted in healthy humans, and one-to-one translation of the results to clinical populations should not be taken for granted, because in neurological and mental diseases, transmitter availability and other features of brain functions might differ from those of healthy humans. Some studies describe that interindividual heterogeneity affects the relationship of exercise and cognitive outcomes (Gomez-Pinilla & Hillman, 2013; Herold, Müller, Gronwald, & Müller, 2019). Studies are needed to explore the reasons for these heterogeneities, accordingly to adapt intervention protocols, including individualization of interventions. Second, previous data show that a single session of aerobic exercise can transiently modulate cortical excitability in the motor cortex regardless of physical activity level (Lulic et al., 2017). Thus, in the present study we did not specifically obtain subjects’ physical activity level. However, this is indeed a limitation to the work, and should be further investigated in future studies. Thirdly, our results show enhanced ICF and diminished SICI after aerobic exercise, which implies that aerobic exercise exerts modulatory effects on both, excitatory glutamatergic neurotransmission and GABA-related inhibition. Nevertheless, since the study did not measure GABA and glutamate concentration directly, the effects of aerobic exercise on these neurotransmitters should be explored in larger detail in future research. Finally, this study was designed to explore the acute effects of aerobic exercise. Long-term effects of aerobic exercise on motor learning and cortical excitability should be investigated in future studies.
ConclusionThis study provides evidence for motor learning performance-improving effects of aerobic exercise in healthy humans. Mechanistically these results can be explained by exercise-caused alterations of cortical excitability, especially a reduction of GABA activity, which might gate learning-related physiology, including plasticity. A more detailed understanding of the mechanistic underpinnings of aerobic exercise effects on motor learning might enable the development of optimal aerobic exercise interventions for various clinical populations. Future studies should address long-term effects of exercise for different exercise parameters and identify directly mechanistic factors linking changes of neurophysiological parameters with cognitive changes as a result of exercise.
Acknowledgements: The study is supported by the Ministry of Science and Technology Taiwan, grant "109-2314-B-002 -030".
Ethics approval: This study was approved by the research ethics committee of National Taiwan University Hospital, Taipei, Taiwan (201911100RIND) and written informed consent was obtained from the participants.