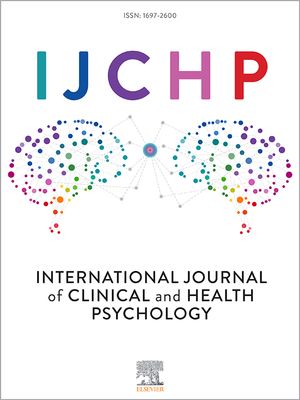
Editado por: Óscar F. Gonçalves
Más datosThe seminal paper of Crick and Koch (2005) proposed that the claustrum, an enigmatic and thin grey matter structure that lies beside the insular cortex, may be involved in the processing of consciousness. As a result, this otherwise obscure structure has received ever-increasing interest in the search for neural correlates of consciousness. Here we review theories of consciousness and discuss the possible relationship between the claustrum and consciousness. We review relevant experimental evidence collected since the Crick and Koch (2005) paper and consider whether these findings support or contradict their hypothesis. We also explore how future experimental work can be designed to clarify how consciousness emerges from neural activity and to understand the role of the claustrum in consciousness.
Consciousness has many components: wakefulness, sentience, awareness, subjectivity, sensation, the sense of free will, and the executive control of the mind. The definition of consciousness has been a controversial topic for centuries, with dozens of theories and countless definitions proposed to explain this complex, yet fascinating, phenomenon (Sattin et al., 2021). Here we will briefly summarize current concepts of consciousness and then consider the possible role of one specific brain area, the claustrum, in generating consciousness (Crick & Koch, 2005).
Psychology-based definitions of consciousnessWhile psychologists have diverse perspectives on consciousness, the most popular theories were proposed by Freud (1915) and Block (1995). Freud's Three Levels of Mind posits that our minds constantly perform at 3 hierarchical levels: conscious, preconscious, and unconscious state (Boag, 2020). According to Freud, the conscious mind contains the sensation, thoughts, working memory, and information that we are presently aware of. It allows us to perform logical reasoning, critical thinking, future planning, and decision-making. The preconscious mind consists of long-term memory, emotion, and imagination which we are not presently aware of but can be easily retrieved into awareness. The unconscious mind is a reservoir of repressed feelings, memories, habits, and desires that are outside of awareness, yet operative in silence (Freud, 1915, 1916).
Block (1995) further differentiated the conscious mind into phenomenal consciousness and access consciousness. Phenomenal consciousness results from the perception of sensory experiences such as hearing, smelling, tasting, and the brain's interpretation of our present environment. Access consciousness is a higher-level process that facilitates reasoning, reflection, and self-awareness which extends beyond the present and mediates control over our actions and speech.
Without delving too deeply into the philosophy of consciousness, there is a consensus in cognitive psychology that consciousness has the following important roles: phenomenal consciousness, namely what a person subjectively experiences, and access consciousness, which is what a person can report and act on. Thus, psychologists typically study consciousness on the basis of such behaviors (i.e., verbal self-reporting and motor actions). In summary, the shared commonality underlying many psychological theories is that consciousness consists of subjective experience (awareness of oneself and the environment) and behavior (the action influenced by this experience).
Neurology-based definitions of consciousnessFrom a neurological perspective, consciousness is typically assessed as a combination of arousal, responsiveness, verbal communication, brain activity, and purposeful movement. The current neurology-based consensus is that consciousness exhibits two key features: (1) the state of consciousness (wakefulness) and (2) the content of consciousness (awareness) (Zeman, 2006; Bayne et al., 2016; Fazekas & Overgaard, 2016).
Wakefulness describes the quantitative degree of consciousness. In a clinical or scientific setting, the degree of consciousness is a continuum of states ranging from full mental alertness and language comprehension to a complete loss of movement in response to painful stimuli. Awareness relates to the qualitative aspects of consciousness, which include complex cognitive abilities such as attention, sensory perception, explicit memory, language, and task execution.
Although there are diverse perspectives and approaches to neurological studies of human consciousness, most consider similar components: (1) waking is the first component in the generation of consciousness, and (2) being aware of the external environment is the second component associated with being conscious. Even though scholars of various disciplines frequently disagree about numerous aspects of consciousness, virtually all agree that to realize consciousness, both wakefulness and awareness are required.
Neural correlates of consciousnessIn “The feeling of life itself”, Christof Koch (2019) wrote, “Consciousness materializes out of the nervous system and endows us with the ability to be aware, have self-knowledge, and hold beliefs about the environment and ourselves.” The phenomenon of consciousness arises from the activity of billions of neurons within our brains. However, the so-called ‘hard problem’ of how conscious experiences - such as emotion, thoughts, and perception - could arise from neuronal circuit interactions is a long-standing puzzle. Many neuroscience-based theories seek to explain consciousness in terms of neural activity occurring within the brain. Here, we summarize three popular theories that attempt to conceptually explain the neural correlates of consciousness (NCC).
Global Workspace Theory – The Global Workspace Theory (GWT) was proposed by Baars (2005). He likened the consciousness contents to a brightly lit spot on the theater stage, while the rest of the dark theater remains unconscious. GWT postulates that mental states only become conscious when they are ‘broadcast’ to a theater within our mind; unconscious content competes for this spotlight to be broadcast (Baars, 2005; Baars, 2017). Dehaene and Naccache (2001) provided an updated “neuronal” interpretation of GWT, the Global Neuronal Workspace. They suggest that conscious cognition emerges from global synchronization, recruitment, and integration involving distributed specialized networks that are mostly unconscious, a position in line with many neuroimaging studies. For example, functional magnetic resonance imaging (fMRI) of the activity of an unconscious brain shows that visual stimuli tend to activate only local regions, such as the visual cortex, while conscious cognition is associated with additional widespread cortical activity, notably toward frontoparietal and medial temporal lobes (Dehaene & Changeux, 2011; Dehaene et al., 2001; Dehaene et al., 2003). Therefore, GWT views consciousness as the product of highly integrated and widespread neural activity.
Integrated Information Theory - The Integrated Information Theory (IIT) posits that the realization of consciousness relies on the physical and functional integration of information within the related neuronal circuitry (Tononi, 2004). Any subjective conscious experience relies on three domains of neural processing: (1) perception of sensory input from external and internal stimuli, (2) decision-making and preparation for actions, and (3) intentional control of thoughts, emotions, motivations, and actions (Brown et al., 2019; Dehaene & Changeux, 2011; Dehaene & Naccache, 2001; Raffone & Pantani, 2010; Zeman, 2001). Thus, these neural domains encode different features of an experience that need to be integrated to generate a unified and coherent conscious representation of our environment.
Neural Synchrony Theory – The Neural Synchrony Theory (NST) proposes that the neural substrate of consciousness integrates varying frequencies of neural oscillations into a coherent pattern within sensory cortices. This integration binds different and overlapping temporal information into a unitary conscious representation. NST suggests that neural synchrony, with precision in the millisecond range, may be crucial for conscious processing (Bastos et al., 2015; Engel & Singer, 2001; Fries, 2015; Varela et al., 2001).
While the mechanisms underlying the GWT, IIT, and NST concepts seem dissimilar, they all share the same general idea: different domains of neural information need to interact to support seamless conscious processing. Thus, NCC-based concepts of consciousness align in suggesting that conscious processing is both unifying and integrative. Thus, the NCC must involve some spatial-temporal integration mechanism that binds multiple domains of information that would otherwise independently encode different facets of the same conscious experience. Given the complexity of such a Herculean task, many wonder if there is a unitary center of consciousness that efficiently coordinates this task.
Any conscious perception is composed of a multitude of sensory modalities (e.g., visual, tactile, auditory, olfactory) and sub-modalities (e.g., color, temperature, motion, pitch). It has long been established that discrete cortical regions are responsible for specialized cognitive functions, for example, the visual cortex, auditory cortex, somatosensory cortex, motor cortex, etc. Although evidence suggests that our perceptual processing is distributed across discrete and far-flung cortices, our perceptions of an experience ultimately become unified.
If the anatomical substrates of perceptual processing are subdivided into functionally and spatially discrete cortical regions, how are these independently processed sensory information ultimately get synthesized into one unified perception? What mechanism mediates the unity of our experience? Understanding the perceptual cognitive binding - how the brain "binds" the activity of different neurons together to produce a coherent representation of the environment – is key to elucidating the anatomical substrates and neural mechanisms of consciousness.
Claustrum and consciousnessThe seminal paper of Crick and Koch (2005) proposed that the claustrum, an enigmatic and thin grey matter structure that lies beside the insular cortex, may be the center of consciousness processing. One of the primary motivations behind their proposal is the extensive and reciprocal interconnectivity of the claustrum with most of the neocortex, as well as subcortical structures (Sherk, 1986; Torgerson et al., 2015; Wang et al., 2017; Zingg et al., 2018; Ham & Augustine, 2022). This is compatible with a global role for the claustrum in integrating information across cortices, as required for an area involved in consciousness. Crick and Koch (2005) proposed that the rhythmic firing of claustral interneurons synchronizes the activities of far-flung cortical populations via gap junctions. Vidyasagar and Levichkina (2019) proposed that the claustrum modulates synchronized neural oscillations between different cortices via cross-frequency coupling between low-frequency claustral oscillations with the high-frequency cortical oscillations. Yin et al. (2016) proposed that the coordination between the claustrum and the striatum mediates temporal-based processing of multisensory integration within consciousness.
In summary, Crick and Koch (2005) proposed that the claustrum may be the center of consciousness, and may contain specialized mechanisms that bind, integrate, and synchronize discrete perceptual, cognitive, and motor information that gives rise to an apparent unity of consciousness. They postulated that the claustrum serves as a hub that integrates the activity of cortical and subcortical neurons to generate a unified and coherent percept. The claustrum is envisioned as the conductor of an orchestra made up of multiple cortical and subcortical members. This metaphor elegantly encapsulates the idea that the claustrum integrates sensory information about different attributes of a single event - both within modalities (e.g., visual color and visual depth) and across modalities (e.g., visual depth and audio source) - into a unitary, coherent conscious percept.
The hypothesis of Crick and Koch (2005) has catalyzed interest in the claustrum, with a notable increase in the number of claustrum-related publications in recent years. Here we discuss experimental evidence published since their paper that either supports or contradicts their hypothesis. Rather than attempting to cover the fuzzier aspects of consciousness (e.g., thought, imagination, motivation, decision-making, etc.), we will focus on the three most fundamental and agreed-upon components of consciousness: wakefulness, awareness, and integration.
Claustrum function in wakefulnessWakefulness is indisputably an integral component of consciousness: awakening is a prerequisite for other elements of consciousness, such as awareness, integration, and much more. Thus, wakefulness is a good place to begin examining the generation of consciousness. Wakefulness is also a measurable state that can be easily quantified and observed, both in humans and in laboratory animals. Therefore, a scientific exploration of consciousness should begin with the awake state.
Clinically, the loss of consciousness (LOC) is defined as an interruption in wakefulness and can often be caused by 3 main reasons: anesthesia, brain lesion, or sleep. In addition, disruption of consciousness is reported to arise from electrical stimulation of the brain. Here we review evidence from these 4 perspectives that suggest the claustrum may serve as a neural substrate of consciousness that potentially correlates with wakefulness.
Anesthesia-induced unconsciousness - Clinicians routinely use general anesthesia (GA) to temporarily switch off consciousness. GA causes LOC, characterized by loss of wakefulness, unawareness, unresponsiveness, amnesia, and immobility. Anesthetics disrupt functional connectivity and synchrony between distant cortices by slowing down neural responses (Munglani et al., 1993) or by impairing inter-cortical interactions (Mashour, 2004). Thus, anesthetics are believed to interrupt consciousness by blocking structures that integrate inter-cortical information (Alkire et al., 2008).
Smith et al. (2017) reported that the functional connections between the claustrum and the medial prefrontal cortex (mPFC) and mediodorsal thalamus (MD) are significantly weakened during isoflurane-induced anesthesia. Similarly, Ollerenshaw et al. (2021) have reported that mouse claustral neurons are silenced during anesthesia. Claustrum neurons express numerous receptors (i.e., α2 adrenergic, kappa-opioid, NMDA, dopaminergic, and GABA receptors) that serve as targets for various anesthetic agents (Chen et al., 2020; Gelegen et al., 2014; Hall et al., 1996; Meoni et al., 1998; Peckys & Landwehrmeyer, 1999). This could potentially explain how anesthetic agents terminate wakefulness via the claustrum. In addition, Pavel et al. (2019) reported that electrical stimulation in the left anterior claustrum induces a transient deepening of anesthesia in rats. While this could provide direct evidence of a role for the claustrum in anesthesia-induced unconsciousness, the small size of the rodent claustrum makes it difficult to rule out the spread of stimulating current to neighboring structures, such as the insula/putamen/striatum.
Taken together, these findings identify functional networks involving the claustrum that are modified in the anesthetized state, suggesting – but not proving - a role for the claustrum in anesthesia-induced unconsciousness.
Lesion-induced unconsciousness – Brain lesions frequently cause LOC and coma in trauma patients and result in the loss of wakefulness, unawareness, unresponsiveness, amnesia, and immobility. Fischer et al. (2016) discovered that lesions of the dorsal brainstem are associated with coma. They reported that the dorsal brainstem is functionally connected to the anterior insula (AI) and anterior cingulate cortex (ACC) in healthy volunteers but is disconnected in patients suffering from disorders of consciousness. This finding aligns with the established role of dorsal brainstem tegmentum in supporting wakefulness and consciousness (Hindman et al., 2018; Magoun, 1952; Parvizi & Damasio, 2003). Based on this, Fischer et al. (2016) proposed that the dorsal brainstem works together with the AI and ACC to drive consciousness. Additionally, they reported that an fMRI activation node detected in the AI extends into the claustrum. Given the limited spatial resolution of fMRI, it is difficult to rule out that the activation node seen in the vicinity of the AI results partially, or even fully, from claustrum activity. Because the AI is only sparsely connected to the ACC (Chia et al., 2017), the claustrum could serve as an intermediary between these two structures. Its proximity to the insula and dense reciprocal connectivity with the ACC and insula make the claustrum an ideal candidate for such an intermediary (Chia et al., 2020).
Snider et al. (2020) reported the activity of the claustrum is anticorrelated with activity in the dorsal brainstem, a region strongly linked to localized lesions that cause LOC. This suggests that the claustrum may play a critical role in impacting consciousness. It is possible that cortical lesions that produce LOC may not map to a single brain region, but instead represent a network involving the dorsal brainstem and the claustrum. Nonetheless, the results suggest that the claustrum plays some role in lesion-induced unconsciousness.
In contrast to the findings above, several other clinical studies report no causal relationship between claustrum lesions and LOC. Duffau et al. (2007) observed no long-term sensorimotor or cognitive impairment and a high rate of full functional recovery in patients with well-delineated, unilateral surgical removal of the claustrum. Likewise, claustral lesions do not always cause LOC and LOC is not uniquely produced by claustral lesions (Chau et al., 2015). Instead, claustral lesions are associated with an increase in the duration, rather than the frequency, of LOC.
Collectively, evidence suggests that the claustrum does not have a crucial and unique role in maintaining wakefulness. It may have some role in supporting wakefulness that requires participation of other brain networks. The findings of Duffau et al. (2007) suggest that the function of each claustrum hemisphere is redundant and can be compensated by the contralateral claustrum. To understand the unique role of the claustrum in wakefulness, future research must determine the unique effects of selective unilateral and bilateral removal (or deactivation) of the claustrum.
Sleep-induced unconsciousness – During a dreamless sleep, consciousness vanishes, and we experience neither wakefulness nor awareness. While sleep is a different state than anesthesia or coma, brain awareness and arousal networks are similarly inactivated (Franks, 2008; Lydic & Baghdoyan, 2005). Because different sleep-wake states are commonly thought to represent different states of consciousness (Laureys, 2005), we will consider the role of the claustrum in regulation of the sleep-wake cycle.
Given the enriched connectivity between the claustrum and both the prefrontal cortex (PFC) and the cingulate cortex (Zingg et al., 2018) - where slow-wave oscillations originate (Massimini et al., 2004; Murphy et al., 2009; Nir et al., 2011) - the claustrum is a promising candidate for regulating slow-wave sleep (SWS). Indeed, recent animal studies provide strong support for claustral involvement in sleep processing: the claustrum has been found to be important for regulation of SWS in reptiles (Norimoto et al., 2020) and in rodents (Narikiyo et al., 2020).
Narikiyo et al. (2020) also demonstrated that optogenetic activation of the mouse claustrum induces global silencing of the neocortex. This suggests that a global synchronization of silent and active states across the neocortex, via slow-wave activity, could regulate the state of consciousness. In addition, patients with claustral lesions exhibit disturbances in global brain activity, which often leads to seizures (Atilgan et al., 2022). This fits with a potential role for the claustrum in regulating cortical excitability and global synchronization of brain electrical activity, which could serve as a mechanism for regulating sleep and wakefulness. Consistent with this concept, patients with claustral lesions evince sleep disturbances (Meletti et al., 2015; Shintani et al., 2000). However, it is unclear whether these are solely the result of claustral lesions or are due to possible confounds, such as medications or concurrent damage to other brain regions.
Sleep is regulated by multiple brain structures, many of which are densely connected to the claustrum (Atilgan et al., 2022). One example is the dorsal raphe nucleus (DRN), the main source of serotonergic input throughout the brain (Monti, 2010, 2011). The claustrum receives extensive input from raphe neurons (Norimoto et al., 2020; Wong et al., 2021; Zingg et al., 2018) and the DRN is essential for the initiation and maintenance of sleep in zebrafish, rats, cats, and mice (Jouvet, 1972; McGinty & Harper, 1976; Monti, 2010; Oikonomou et al., 2019). The claustrum also expresses many serotonin receptors (Gehlert et al., 1991; Koyama et al., 2017; Morales et al., 1998; Norimoto et al., 2020; Wong et al., 2021). Results from genetic, neurochemical, electrophysiological, and neuropharmacological studies have suggested that serotonin is involved in regulation of sleep-wake cycles (Monti, 2011; Ursin, 2002; Watson et al., 2010). Electrophysiological results have shown that serotonin exerts a net inhibitory effect on the claustrum (Wong, 2021; Wong et al., 2021). Taken together, it is plausible that the claustrum regulates sleep-wake states via serotonergic inhibitory inputs from the DRN.
The neocortex and hippocampus are activated during rapid eye movement (REM) sleep through ascending projections from the claustrum and the supramammillary nucleus (Renouard et al., 2015). Following REM sleep, claustral neurons connected to frontal areas (such as the ACC) exhibit increased expression of Bdnf, FOS, and ARC genes; these are markers for neural activation and synaptic plasticity, suggesting that the claustrum is also more active during REM sleep. This could indicate a role for the claustrum in REM sleep, though these results are also consistent with the idea that claustral projections to the neocortex and hippocampus have a role in memory consolidation or dreaming, rather than regulating REM sleep per se.
Stimulation of the neocortex (Kuki et al., 2013; Vyazovskiy et al., 2009) and the thalamus (David et al., 2013) have also been reported to elicit slow-wave cortical activity in rats. In humans, similar slow-wave activity can be induced using brain-wide stimulation via transcranial magnetic stimulation (Massimini et al., 2007). Therefore, the claustrum may not have unique or global control over SWS. Instead, the claustrum may function as a component of a sleep-regulatory network that modulates SWS or REM but does not necessarily initiate or inhibit the sleep rhythm (Norimoto et al., 2020). Further research will be required to establish the precise role of the claustrum in sleep and wakefulness.
Stimulation-induced alteration in consciousness - The role of the human claustrum in consciousness has been probed via electrical stimulation. Koubeissi et al. (2014) reported the remarkable finding that stimulation near the claustrum of one epilepsy patient elicited a complete and reversible disruption of consciousness, including arrest of volitional behavior, unresponsiveness, and amnesia. However, a follow-up study failed to replicate this finding in five other epilepsy patients whose claustrum was electrically stimulated, either unilaterally or bilaterally (Bickel & Parvizi, 2019).
This contradiction probably arises from confounds in the experiment of Koubeissi et al. (2014). Firstly, it is unclear whether the stimulating current reached the claustrum; in their experiments, the stimulating electrode was placed near, but not within, the claustrum. Secondly, the electrical current applied during stimulation was greater in both magnitude and duration than that used by Bickel and Parvizi (2019). Strong stimulation current could potentially flow to cortical neurons, resulting in direct and widespread cortical silencing that disrupts normal awareness processing, independent of the claustrum (Atilgan et al., 2022). Thirdly, the patient had previously undergone an ipsilateral temporal lobectomy which may have contributed to the observed disruption of consciousness.
In summary, the predominance of claustral stimulation results do not support the hypothesis of Crick and Koch (2005). Although it is challenging to assess consciousness in animal models, it would still be useful to replicate these experiments in mice by using optogenetic approaches to control claustrum activity more precisely and unambiguously.
Claustrum role in awarenessA second integral component of consciousness is awareness of one's self and their surroundings. The perceptual awareness of sensory stimuli to generate a unified conscious percept, is the foundation of the binding and integration theory of Crick and Koch (2005). Here we discuss the potential involvement of the claustrum in awareness: this includes salience, attention, and altered states of awareness.
Salience and attention - Attention is categorized into 2 distinct pathways: bottom-up and top-down. Bottom-up attention is passive guidance of involuntary awareness driven by perceptual salience (i.e., salient stimuli that possess novel or prominent properties relative to the background). Top-down attention is a voluntary process that directs awareness onto information based on internally guided contexts (Katsuki & Constantinidis, 2014). The content of awareness is highly dependent on the attended subject (Freud, 1916). Hence, many are convinced that perceptual salience and attention are prerequisites for awareness and, thus, consciousness (Cohen et al., 2012; Nani et al., 2019; O'Regan & Noë, 2001; Posner, 1994; Sikkens et al., 2019; Simione et al., 2019).
Proposed roles of the claustrum include perceptual salience (Smith et al., 2019) and attentional modulation (Goll et al., 2015). The claustrum is hypothesized to detect salient sensory changes and then recruit the attentional network to process the salient signal. Given the quiescent nature of claustral neurons and how these neurons respond transiently to sudden stimuli (Remedios et al., 2014), the claustrum could serve as a salience detector. Day-Brown et al. (2017) proposed that local GABAergic circuits normally inhibit claustral activity, which is overcome transiently by excitatory cortico-claustral circuits to signal a salient stimulus. Such GABAergic circuitry, as well as claustral connectivity with sensory and frontal cortices (Goll et al., 2015; Mathur, 2014; Chia et al., 2020), fit with a possible role for the claustrum in gating attention.
To date, empirical evidence of a salience detection function for the claustrum is lacking. The most relevant evidence comes from measurement of responses of the macaque claustrum to acoustic stimulation: the claustrum responds to the onset of sound (Remedios et al., 2014). Claustrum responses are stronger, with shorter latencies, to novel sound stimuli with higher signal-to-noise ratio.
Claustral neurons are also more active when rats explore a novel object in the environment and are significantly less active when the object is removed (Jankowski & O'Mara, 2015). Claustral activity dynamically follows the introduction, removal, repositioning, and location change of the novel object. These neurons are active in both light and dark conditions, suggesting that the claustrum is co-activated by both somato-sensory and visual inputs.
Because the claustrum preferentially projects to the ACC (Smith & Alloway, 2010; Chia et al., 2017; Chia et al., 2020), an area known to be involved in selective attention (Pardo et al., 1990; Weissman et al., 2005; Totah et al., 2009; Schneider et al., 2020), the claustrum could receive top-down attentional influences from the ACC. Indeed, claustrum neurons can transiently amplify inputs from the ACC (White & Mathur, 2018). During successful completion of an attentional task, the activity of ACC neurons projecting to the claustrum is elevated (White et al., 2018), while optogenetic inhibition of these neurons disrupts performance accuracy during attentional tasks (White & Mathur, 2018). In addition, extensive functional connectivity is observed between the claustrum and the ACC during cognitive tasks (Krimmel et al., 2019). Therefore, it is plausible that the claustrum and the ACC work together during an attention-requiring cognitive task.
A role for the claustrum in attention has been more directly supported by several other studies. Claustral inhibition renders mice more susceptible to auditory distraction and disrupts performance during maternal pup retrieval and two-alternative-forced-choice task (Atlan et al., 2018). In addition, optogenetic activation of claustral neurons decreases cortical responses to pure sound tones, suggesting that claustrum activity may suppress encoding of task-irrelevant auditory stimuli (Atlan et al., 2018). This suggests that a subset of claustral neurons may suppress auditory distractions during attentional tasks.
Partial claustral inactivation results in delayed acquisition of conditioned motor learning in rabbits (Reus-García et al., 2020). It is plausible that such inactivation produced deficits in attentional and salience processing that are required during associative learning, thereby yielding poorer learning. Further, a subset of claustral neurons are active in response to combined auditory-somatosensory stimuli, but not to unimodal, task-irrelevant stimuli (Reus-García et al., 2020). Because this response pattern lasts only until the completion of conditioned motor learning, it is possible that once-salient, task-relevant stimuli lose their perceptual salience after repeated exposures.
In addition, the claustrum is apparently involved in the formation of mPFC cell assemblies required for attentional set-shifting. Chemo-genetic inhibition of the claustrum disrupts this formation and subsequent behavioral task accuracy, suggesting that the claustrum-mPFC network is important for attentional set-shifting (Fodoulian et al., 2020).
On the other hand, chemogenetic activation of the claustrum is reported to disrupt attention and significantly attenuate task accuracy (Liu et al., 2019). This could indicate that the claustrum modulates attention negatively, though it is also possible that the disruption of attention results from an abnormal pattern of claustrum activity induced by the chemogenetic activation.
The ACC has been implicated in complex consciousness, for example playing a role in the sense of free will (Darby et al., 2018). Crick (1995) proposed that the ACC is the center of free will. Robust activity is observed in the ACC and the insular-claustrum region during the suppression of blinking, which is a forceful action that relies on free will to resist the natural urge to blink (Lerner et al., 2008). In this context, the claustrum-ACC network could be activated by attention to a task and then recruit circuitry required to maintain eye opening. Thus, claustrum-related networks could be involved in sub-components of consciousness, in particular salience or attention.
Altered states of awareness - Conscious awareness can be altered by psychoactive substances, mental disorders, or mental practices (Revonsuo et al., 2009; Manuello et al., 2016). During an altered state of awareness (ASA), an individual experiences a state of awareness that does not resonate with reality (e.g., hallucinations, delusions, memory distortions, etc.). Here we review evidence that associates the claustrum with ASA.
Inhibition of the claustrum, by activating κ-opioid receptors (KOR), has been proposed to underlie the mind-altering effects of Salvia divinorum, which is a potent dissociative hallucinogen (Stiefel et al., 2014). The active ingredient in Salvia divinorum is Salvinorin-A, which is a KOR agonist. Consistent with this proposal, KOR are densely expressed in the claustrum (Chen et al., 2020). The claustrum also densely expresses serotonin receptors (Wong et al., 2021). Claustral neurons are activated by a psychedelic compound, 2,5-Dimethoxy-4-iodoamphetamine (DOI), which is an agonist of serotonin receptors (Martin & Nichols, 2016). Another serotonin receptor agonist, psilocybin, is a hallucinogen that selectively decreases claustral activity without affecting neighboring structures such as the insula or putamen (Barrett et al., 2020). Psilocybin induces widespread dysregulation of cortical activity and alters the functional connectivity between the claustrum and the default mode network and the frontoparietal task control network. Hence, ASA could arise from either claustral activation or inhibition, via differing modes of psychedelic drug action. Much more work is needed to elucidate the actions of psychedelics on the claustrum and, more generally, to understand the role of the claustrum in ASA.
Individuals with severe mental disorders, such as schizophrenia, often experience altered awareness and interpret reality differently. The volume of the claustrum is smaller in schizophrenic patients (Bernstein et al., 2016). The hallmark signs of schizophrenia - delusions and hallucinations – are associated with a decrease in the intrinsic functional connectivity between the claustrum and the dorsal ACC (Jacobson McEwen et al., 2014). Further, the gray matter volumes of the claustrum and insula are inversely correlated with the severity of delusions and hallucinations (Cascella et al., 2011). Ishii et al. (2011) have reported that symmetrical claustral lesions caused by viral encephalitis are associated with temporary episodes of delusion and hallucinations. Other studies have similarly reported altered mental states - typically confusion, disorientation, disordered perception, stupor, delusion, and hallucination - in patients suffering from claustral lesions (Atilgan et al., 2022). Schizophrenia is associated with hypersensitivity to non-salient stimuli (Roiser et al., 2012; Shitij Kapur, 2003) and deficits in sensory gating (Hall et al., 2010). Supporting the salience-processing role of the claustrum, Bak et al. (2011) found that activity in the claustrum is linked to normal sensory gating. It is possible that claustral disruption impairs normal sensory gating in the brain to filter out irrelevant, non-salient stimuli to minimize sensory overload. In the case of schizophrenia, this constant sensory overload may contribute to the psychotic symptoms (Braff & Geyer, 1990; Patterson et al., 2008).
In summary, although current evidence suggests that the claustrum is involved in salience and attention, and that modified claustral activity can alter the integrity of conscious awareness and perception, the precise mechanisms involved remain to be elucidated.
The claustrum and multisensory integrationWe experience consciousness as a unified representation, rather than discrete perceptions of sight, sound, smell, emotion, etc. The claustrum receives afferent inputs from many sensory cortices, motivating the integrative theory of Crick and Koch (2005). Further, Smith and Alloway (2014) have suggested that the claustrum is engaged when multisensory stimuli are perceived altogether and when multisensory integration is required for behavior. Such ideas are consistent with observations that single-mode, task-irrelevant stimuli rarely activate the claustrum, while claustral activity is increased by presentation of multisensory stimuli (e.g., paired auditory and tactile stimuli) (Reus-García et al., 2020). Similarly, the claustrum is minimally responsive towards passive sensory representation but strongly responsive during behavior that requires sensory-motor integration (Ollerenshaw et al., 2021).
The rat claustrum is involved in interhemispheric coordination of the primary somatosensory (S1) and motor (M1) cortices during whisking and exploratory behavior, suggesting that it plays a role in coordinating sensory-motor cortical activity across hemispheres (Smith et al., 2012). Furthermore, a subset of rat claustral neurons selectively respond to spatial location, boundaries enclosing a space, and to novel objects, both in light or dark conditions (Jankowski & O'Mara, 2015). This suggests that the claustrum could concurrently respond to multisensory stimuli (i.e., tactile and visual). Collectively, these findings support a role for the claustrum in integrating multisensory information, primarily during cognitive attention and sensory-motor integration.
However, there is evidence arguing against this hypothesis. In the macaque claustrum, individual claustral neurons reportedly respond to either auditory or visual stimuli, but not to both (Remedios et al., 2010). Because the response to one modality was not modulated by another modality, this suggests the lack of multisensory integration within the claustrum. Further, while the rat claustrum projects to both S1 and M1, the S1 does not project back to it (Smith et al., 2012). Thus, the claustrum is unlikely to be the integrator for somatosensory and motor cortical information. In summary, while these findings indicate the multisensory nature of the claustrum, they argue against a multisensory integrator role for the claustrum.
To reconcile the conflicting experimental evidence regarding multimodal integration within the claustrum, Smythies et al. (2014) proposed that when connected cortical areas involved in the same task begin to synchronize their activities, their connections to the claustrum generate intra-claustral neural synchrony. The claustra then enhance the synchrony between these connected cortical areas through their efferents back to the respective cortical targets. While speculative, this hypothesis can reconcile existing experimental data and warrants further investigation.
Taken together, these findings support the theory for the claustrum to process sensory-motor representations, as well as to coordinate multi-sensory activity required in cognitive attention and sensory-motor tasks. However, they argue against a central integrative function because those representations do not appear to be integrated within the claustrum. Therefore, current information does not definitively validate or disprove a role for the claustrum as an integrator for consciousness.
Is the claustrum involved in consciousness?Although our understanding of the neural substrates of consciousness is expanding, it remains imprecise (Lenharo, 2023). The previous sections describe evidence suggesting that the claustrum may play roles in wakefulness, awareness, and integration. These are consistent with a role for the claustrum in consciousness. However, many other brain areas beyond the claustrum undoubtedly also are involved in consciousness. Among sub-cortical structures, the thalamus, basal ganglia, and hippocampus are most often implicated, whereas the cingulate, prefrontal, and temporal areas are the most relevant cortical structures (Sattin et al., 2021).
A traditional view is that consciousness is supported by the neurons within the ascending reticular activation system (ARAS), which plays a crucial role in sustaining arousal and wakefulness (Englot et al., 2017; Jang & Lee, 2015; Jones, 2003). The paraventricular nucleus of the thalamus (PVT) has also been identified as a key node for controlling the state of awakening and arousal (Groenewegen & Berendse, 1994; Ishibashi et al., 2005; Kirouac, 2015; Nascimento et al., 2008; Ohno & Sakurai, 2008; Van der Werf et al., 2002). The claustrum is known to map to nuclei affecting sleep and sustaining wakefulness, such as the DRN (Norimoto et al., 2020), PVT (Zingg et al., 2018), and possibly ARAS (Fischer et al., 2016). However, while wakefulness sustained by ARAS and PVT is necessary, wakefulness alone is insufficient for conscious awareness.
Awareness is thought to arise within the neocortex. Conscious awareness may emerge from the frontal lobe, including the prefrontal and central anterior cortex (Blake & Logothetis, 2002; Breitmeyer & Ogmen, 2000; Francis, 2000; Imamoglu et al., 2012; Tsuchiya & Koch, 2005). Alternatively, it could arise in the hindbrain, including the occipital/parietal and central posterior cortical regions (Bianchi & Sims, 2008; Boly et al., 2017; Koch et al., 2016; Merker, 2007; Seth, 2018). Experimental results are often contradictory, likely due to differences in techniques used. Classic report-based paradigms reveal broad fronto-parietal cortical activation during visual–motor tasks that contrast perceived versus unperceived stimuli (Dehaene & Changeux, 2011). However, this may reflect processes that are merely a prerequisite for conscious awareness — such as vigilance, attention, expectation, task preparation, and reporting — rather than the conscious experience itself (Aru et al., 2012; de Graaf et al., 2012; Koivisto & Revonsuo, 2010; Miller, 2014). Newer no-report paradigms (Tsuchiya et al., 2015), which do not require subjects to produce an overt report, identify a more restricted and consciousness content-specific activation in the posterior cortical areas (Frässle et al., 2014).
It is likely that consciousness requires multiple components working together seamlessly; this includes cooperation between multiple cortical and subcortical regions, each of which serves as an island of consciousness. Elaborating on the conductor metaphor (Crick & Koch, 2005), it appears that the orchestra requires several conductors that work together to support and manage consciousness. If this is the case, it will be important to identify the mechanisms or structures responsible for such coordination (Bayne et al., 2020). The relationship between the claustrum and these consciousness-related areas is unclear. Evidence summarized here indicates that the claustrum participates in large-scale networks that subserve functions such as wakefulness, sleep, and attention. These are important components of consciousness. The claustrum is also hypothesized to mediate synchronization and modulation of cortical functional networks to integrate and unify our conscious percept.
The claustrum is often implicated in selective attention (Goll et al., 2015) and projects to the ACC, a structure known to be involved in attention (Chia et al., 2017; Krimmel et al., 2019; White & Mathur, 2018; White et al., 2018). Because attention strongly influences our conscious awareness, perhaps the claustrum contributes to consciousness merely as an attentional hub. In this role, it would redirect attentional traffic across the neocortex and retrieve memories into conscious awareness.
Recently, Budson et al. (2022) proposed an alternative theory based on the concept that we do not experience our perceptions directly, but instead experience them by recalling a memory of our perceptions. According to this theory, a majority of our decisions and actions happen unconsciously; our awareness of these decisions and actions only occurs later, in the form of recalling a memory. This aligns with observations of sensorimotor systems in the primate brain that function in the absence of consciousness, termed the “zombie mode” by Koch and Crick (2001). These are stereotypical, routine, rapid, and effortless actions that outpace consciousness. This is particularly true of the highly practiced and ritualized sensorimotor activities, such as playing musical instruments, using sports skills, or dancing. We become conscious of the actions of our zombies, but usually only retrospectively by remembering our actions.
A prime example of zombie-mode behavior is sleepwalking. A sleepwalker is neither awake nor aware (Jacobson et al., 1965; Schenck et al., 1998; Zadra et al., 2004), yet can carry out complex movements such as talking, walking, eating, cleaning, cooking, and even driving a vehicle (Popat & Winslade, 2015). Sleepwalkers are not conscious, because they neither experience sensory perception nor have any memory of the incident. Not only does this challenge our deep-seated belief that consciousness is necessary for performing complex behavior, but it also reveals how our locomotor control system can be automated and dissociated from our consciousness during sleep and waking state (Kannape et al., 2017). Further, the onset of cerebral activity for a voluntary motor act precedes the conscious intention to act by several hundred milliseconds, challenging the initial assumption that conscious urge is needed to initiate a voluntary act (Libet et al., 1983). This suggests that cerebral initiation of a voluntary movement can begin unconsciously before there is any awareness about the intention to act.
Koch and Crick (2001) proposed that consciousness arises to create explicit memory and to plan future actions, opening a potentially infinite behavioral repertoire. Budson et al. (2022) proposed that consciousness enables us to store, recall and recombine memories to guide future actions. Thus, our conscious percept could be a creative combination of sensory memory, working memory, episodic memory, semantic memory, etc.
It has long been established that discrete cortical regions are responsible for specialized cognitive functions. Budson et al. (2022) suggests that each cortex autonomously behaves like an island of consciousness. Each cortex contributes to the formation of sensory or working memory within its specialized domain (Fig. 1). For example, (A) motor cortex processes awareness awareness and memory of motor action, (B) prefrontal cortex processes working memory; (C) Broca's area processes awareness and memory of speech, (D) somatosensory cortex processes awareness and memory of somatic information, (E) auditory cortex processes auditory awareness and memory, and (F) visual cortex processes visual awareness & memory.
Hypothetical cortical islands of consciousness: (A) primary motor cortex; (B) ACC/PFC (Attention or working memory); (C) Broca's area (Verbal speech/report); (D) Somatosensory cortex; (E) Auditory cortex; and (F) Visual cortex.
ACC: anterior cingulate cortex, CLA: claustrum, PFC: Prefrontal cortex
Figure modified from Zhao et al. (2019).
Given its tiny size, small number of neurons, and quiescent nature, it is unlikely that the claustrum has sufficient computational bandwidth to enable the continuous stream of information processing associated with consciousness. Coordinating and redirecting “attentional traffic” toward memories across islands of consciousness may be better suited to the computational capacity of the claustrum. If memories are packaged and stored as readily accessible units within cortical islands, then retrieving the memories would be simpler and faster than constantly integrating new information. Rather than integrating and synthesizing new content, a claustrum-mediated attentional network could simply retrieve a memory and bring it into conscious awareness. Rather than perceiving the world and our actions in real time, such a system would let us remember our perceptions and actions in retrospect.
The claustrum has also been implicated in REM sleep (Fenk et al., 2023; Renouard et al., 2015), which has been hypothesized to consolidate memory (Boyce et al., 2017). When multiple sensory and working memories are repeatedly accessed together, they could be consolidated and woven into a bundled and time-stamped unit during REM sleep. These bundles could then be stored as long-term episodic memory, procedural memory, or semantic memory and could be easily retrievable via a claustrum-mediated attentional network.
Such a consciousness-enabling memory system could potentially explain the apparent unity of consciousness. The claustrum is well-connected to the ACC (attention), PFC (working memory), sensory cortices (sensory memory), motor cortex (motor/procedural memory), amygdala (fear-related memory), hippocampus (spatial/episodic memory), entorhinal cortex (episodic/contextual memory), and retrosplenial cortex (spatial memory) (Atlan et al., 2017; Brennan et al., 2021; Jackson et al., 2020; Kitanishi & Matsuo, 2017; Miller et al., 2014; Mitchell et al., 2018; Torgerson et al., 2015; Zingg et al., 2018). Via such extensive connectivity, the claustral projections could simultaneously retrieve memory units from many cortical islands. When memory units that correspond to multiple facets of a singular event (e.g., visual, audio, and scent memories) concurrently enter the conscious awareness, it could appear as a unified representation.
Challenges, limitations, and future directionsUnderstanding consciousness is a complex problem. Our limited current knowledge of consciousness makes it difficult to consolidate all perspectives. There are many definitions of consciousness, a multitude of viewpoints, and multifaceted approaches to studying consciousness. Many aspects of consciousness remain subjective. For example, are anesthesia and sleep the same form of unconsciousness? Assessment is often controversial because aspects of consciousness are difficult to quantify, e.g., are we directly measuring the degree of consciousness or indirectly observing a consciousness-related phenomenon? Because consciousness is not accessible to outsiders, researchers typically rely on self-reporting to assess the degree of consciousness. These “measures” of consciousness vary considerably from individual to individual, trial to trial, and study to study. An important direction for future research will be to develop a more objective measure of consciousness in humans.
The synthesis of knowledge about relationships between the claustrum and consciousness is complicated by several factors, including (1) the relative scarcity of studies that focus on the claustrum, (2) the lack of spatial resolution in fMRI to unmistakeably delineate the claustrum from neighboring structures and to disentangle claustrum activity from that of neighboring areas, such as the insula and striatum; and (3) the lack of a clear understanding of the general role of the claustrum in behavior. As mentioned earlier, there is an upward trajectory in the amount of claustrum-related research, so we are hopeful that factor (1) will be overcome in the foreseeable future. This, in turn, will enable better understanding of claustral function (factor 3). Factor (2) will require greater use of 7T MRI instruments (Krimmel et al., 2019) and better segmentation algorithms (Li et al., 2021).
Furthermore, clinical results related to claustrum function are confusing and inconsistent: diverse signs and symptoms have been reported in response to claustrum dysfunction or experimental stimulation. Patients experience varying levels and types of disturbance in cognitive function, perceptual abilities, mental state, electrical activity, or sleep. This is further complicated by the fact that bilateral lesions that are restricted to the claustrum are exceedingly rare; lesions in this area typically include damage to the surrounding white matter. Often, unilateral claustral lesions occur and studies do not account for possible compensatory functions of the other claustrum. As mentioned previously, understanding the role of the claustrum in wakefulness requires elucidation of the effects of selective unilateral and bilateral removal (or deactivation) of the claustrum. In sum, more carefully controlled clinical research into claustrum function is warranted.
In order to understand the role of claustrum in awareness, future research could aim to clarify how the claustrum mediates sensory gating and modulates attentional switching, to prevent sensory overload in schizophrenic patients versus healthy subjects. In addition, to understand the role of the claustrum in altered awareness more work will need to be done to determine the actions of psychedelic agents on the claustrum.
To date, claustrum stimulation studies of consciousness have largely been done in humans. Although it is challenging to assess consciousness in animal models, it would still be useful to replicate such claustral stimulation experiments in mice by using optogenetic approaches to control claustrum activity more precisely and unambiguously.
The main limitation of studying claustrum function in animal models is the small size, irregular shape, and anatomical location of the claustrum. These anatomical constraints make precise surgical removal and pharmacological intervention extremely difficult. Even with state-of-the-art technology, such as optogenetics (Mahmoudi et al., 2017) and chemogenetics (Campbell & Marchant, 2018), in vivo functional manipulation and specific targeting of claustral neurons remain arduous tasks. While there has been progress in identifying genetic markers that allow semi-selective expression of transgenes in claustral neurons but not in adjacent regions (Mathur et al., 2009; Watakabe, 2017; Watakabe et al., 2014), currently there is no gene promoter known that is entirely selective for claustrum neurons. Such refinements should be paired with rigorous optimization of positioning when using imaging, measurement, and manipulating tools. Further, to take advantage of such improvements in manipulating the claustrum of animal models, it will be crucial to develop an experimental paradigm for assessing consciousness in animal models.
Understanding the role of claustrum in integration might benefit from the alternative theory of consciousness-enabling memory system. The claustrum could act as a hub to redirect attentional traffic across various cortical islands and retrieve consciousness-related content in the form of memories. Testing this theory will require analysis of how memories are processed in various cortical regions and determining whether claustral projections have access to these memories. Thus, future research should aim to elucidate the relationship between the claustrum and brain structures that are involved in memory formation, retrieval, and consolidation.
Finally, if the claustrum is indeed integrating information both within and across modalities, it is unlikely that bilateral claustral silencing would interfere with “zombie mode” sensory-motor behaviors, such as instinctual, routine, and stereotyped behaviors evoked by basic sensory input. To circumvent such complications, future behavioral analyses of claustrum function should be based on tasks with higher cognitive workload (e.g., involving multisensory stimuli within or across modalities).
ConclusionsThe hypothesis of Crick and Koch (2005) has catalyzed interest in the claustrum in the search for neural correlates of consciousness. We have summarized theories of consciousness, reviewed the relationship between the claustrum and consciousness, and examined experimental evidence that either supports or contradicts the hypothesis that the claustrum is the seat of consciousness. We have also explored an alternative theory about consciousness and discussed how future experiments could investigate the role of the claustrum in consciousness.
Given what we now know, the most parsimonious explanation is that the claustrum serves as a structural interface between awakening, awareness, and integration, the 3 fundamental components of consciousness. The key roles of the ARAS and PVT in arousal and awakening could enable consciousness, with cortical islands serving as storage sites for awareness content, while the claustrum may be responsible for retrieval of such content across various cortical islands (Fig. 2). To determine how the claustrum could coordinate this collection of brain areas subserving different functions, further experiments certainly will be needed.
Illustration of the possible neural network for consciousness. The paraventricular nucleus of the thalamus (PVT) is in control of awakening and arousal. The neurons in the posterior cerebral cortex are found to be involved in awareness and generation of consciousness content. The claustrum may act as the attentional hub for coordinating information transmission across cortical islands.
CLA, claustrum; PVT, paraventricular; Th, thalamus; NCC, neural correlates of consciousness.
Figuremodified from Zhao et al. (2019).
We hope that this review will inspire future research that explores claustral function from a perspective that clarifies the relationship between the claustrum and brain structures typically involved in memory formation, retrieval, and consolidation, as well as the relationship between the claustrum and structures involved in sleep, arousal, and wakefulness.
CRediT authorship contribution statementYin Siang Liaw: Conceptualization, Writing – original draft. George J. Augustine: Funding acquisition, Writing – review & editing.
This work was supported by research grant MOE2017-T3-1-002 from the Singapore Ministry of Education.