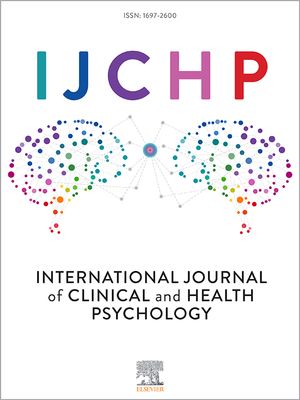
Editado por: Óscar F. Gonçalves
Más datosUnderstanding the mechanisms underlying human consciousness is pivotal to improve the prognostication and treatment of severely brain-injured patients. Consciousness remains an elusive concept and the identification of its neural correlates is an active subject of research, however recent neuroscientific advances have allowed scientists to better characterize disorders of consciousness. These breakthroughs question the historical nomenclature and our current management of post-comatose patients.
MethodThis review examines the contribution of consciousness neurosciences to the current clinical management of severe brain injury. It investigates the major impact of consciousness disorders on healthcare systems, the scientific frameworks employed to identify their neural correlates and how evidence-based data from neuroimaging research have reshaped the landscape of post-coma care in recent years.
ResultsOur increased ability to detect behavioral and neurophysiological signatures of consciousness has led to significant changes in taxonomy and clinical practice. We advocate for a multimodal framework for the management of severely brain-injured patients based on precision medicine and evidence-based decisions, integrating epidemiology, health economics and neuroethics.
ConclusionsMajor progress in brain imaging and clinical assessment have opened the door to a new era of post-coma care based on standardized neuroscientific evidence. We highlight its implications in clinical applications and call for improved collaborations between researchers and clinicians to better translate findings to the bedside.
Acquired brain injury is a neurological condition with a major impact on healthcare systems (Dewan et al., 2019; James et al., 2019). As we start to better understand the implications of mild brain injuries and concussions on long-term cognitive functions and neural plasticity (Bashir et al., 2012; Haarbauer-Krupa et al., 2021), severe brain injuries remain a substantial burden on patients as they often have irreversible consequences on their quality of life (Iaccarino, Carretta, Nicolosi, & Morselli, 2018). Before the invention of mechanical ventilation, the evolution of patients with severe brain injury was traditionally divided into binary outcomes: those who could not spontaneously sustain the necessary vital functions to survive the acute phase and those who showed sufficient preservation of brainstem structures to sustain respiratory activity. With the development of mechanical ventilation in the 1950s, the ability to maintain patients alive using artificial measures became a reality, and the family of disorders of consciousness (DoC) was born (Fins, 2019). This new group of diseases introduced a dimension of ethical considerations as the decision to preserve basic vital body functions became a human call (Young et al., 2021). A wide array of specific procedures and supportive techniques needed to be developed and refined to create a framework for the care of these patients, from the acute stage in the intensive care to the recovery of daily life activities. Notably, the establishment of accurate methods to evaluate the level of consciousness in these patients and monitor their improvements rapidly became a cornerstone of coma science. As it became clear that only a fraction of these patients were able to recover functional independence, therapeutic decisions required careful ponderation of prognostic uncertainty and unequivocal recovery endpoints. To homogenize the nonspecific and redundant nomenclature previously used, a unified diagnostic classification for DoC was progressively established in the last 50 years and is still constantly evolving (Zasler, Aloisi, Contrada, & Formisano, 2019). Initially based on bedside clinical signs and behaviors that would denote the presence or absence of consciousness, this taxonomy is starting to include measures derived from neurophysiology and neuroimaging (Edlow et al., 2017; Schiff, 2015; Thibaut et al., 2021). Despite these technological advances in our understanding of consciousness and its impairments, there is still an active debate on the clinical signs warranting the presence of conscious awareness or the minimal brain activity requirements to maintain consciousness (Walter, 2021). The ever-changing state of the science and the unresolved conceptual debates on neurophysiological substrates pose major challenges to researchers and clinicians alike (Giacino, Fins, Laureys, & Schiff, 2014). Yet, recent European and American guidelines on the diagnosis of DoC are paving the way towards global and evidence-based post-coma care (Giacino et al., 2018; Kondziella et al., 2020)
The definition of consciousness itself is still actively debated among the scientific community up to today (Fabbro, Cantone, Feruglio, & Crescentini, 2019). The subjective nature of the conscious experience is inherently associated with a difficulty to define common outcomes. By definition, consciousness is private to the organism experiencing it and requires a transformative step to observe it through the experimental lens (Butler, 2012). Additionally, its high-order cognitive nature limits the translational potential of animal studies on consciousness, as few conscious human behaviors can be reliably paralleled in animals (Paul, Sher, Tamietto, Winkielman, & Mendl, 2020). Scientists have used several conceptual frameworks to objectify the conscious percept, an array of mechanistic theories aiming to explain its generation have been formulated, which further complicate the communication process and the interpretation of findings (Northoff & Lamme, 2020).
For clinicians, providing optimal clinical care to patients with DoC is challenging for several reasons. First, the diversity of consciousness assessment methods is a hurdle to overcome. Diagnosis is currently based on repeated and standardized bedside assessment, which is time-consuming and requires appropriate training (Seel et al., 2010; Wannez, Heine, Thonnard, Gosseries, & Laureys, 2017). Additionally, existing scales do not account for newly identified signs of consciousness or brain activity measures (Fitzpatrick-DeSalme, Long, Patel, & Whyte, 2022; Mat et al., 2022). The lack of consensual diagnostic guidelines or iterative decision trees including neuroimaging exams is a major setback (Sanz, Thibaut, Edlow, Laureys, & Gosseries, 2021). Second, our limited understanding of the pathophysiology underlying consciousness alterations has further countered the availability of evidence-based treatments tailored to the disruption of specific neural functional networks or neurotransmitter pathways (Edlow, Claassen, Schiff, & Greer, 2020). Finally, the heterogeneity of patient profiles precludes the use of a one-size-fits-all therapeutic approach, as individual patients may respond to specific therapies. These confounding factors can lead to suboptimal clinical management and marginalization of this already vulnerable population.
The present review explores the contribution of recent advances in consciousness research to the clinical landscape of patients with DoC, in terms of diagnosis, prognosis, treatment, but also epidemiology, economics and ethics. It aims to highlight how an improved understanding of conscious processes and biological signatures of consciousness can contribute to build a modern framework for the care of brain-injured patients, based on reliable evidence.
A scientific approach to consciousnessA central obstacle in the development of a unified framework to study consciousness has been the lack of consensus on its very definition. The abstract nature of the term and the various concepts it has been used for throughout history contribute to its elusive and multifaceted representation. The cognitive psychologist George Miller, who was a pioneer of the information processing theory, declared that “consciousness is a word worn out smooth by many tongues. Depending upon the figure of speech chosen it is a state of being, a substance, a process, a place, an epiphenomenon, an emergent aspect of matter, or the only true reality” (Miller, 1962). Indeed, the essence we choose to give to this concept is a matter of arbitrary definition. To study DoC, scientists have preferred to focus on the neurological deficits and absent features that can be observed in severely brain-injured patients, to define consciousness as a photographic negative, by the outlines of its missing components, rather than formulated as a positive phenomenon that we struggle to define. The functional “default mode network”, defined as a collection of functionally connected brain areas when a subject is conscious and at rest, is a good example of how consciousness has been considered as a default function of rest in this field of research (Guldenmund, Vanhaudenhuyse, Boly, Laureys, & Soddu, 2012).
Breaking down a biological complex process into simpler components can be helpful to disentangle and study its physiology. A widely used framework to apprehend consciousness has split its nature into two fundamental axes: arousal and awareness (Laureys, 2005). In this model, arousal is defined as the level of vigilance exhibited by the patient, regardless of the content of thought. At the bedside, it can be assessed by the opening of the eyes, either spontaneously or following stimulation. It is therefore recovered rather early in the spectrum of DoC as all diagnostic categories above coma require the presence of eye-opening. On the other hand, the awareness component is recovered more progressively in the course of rehabilitation. It is commonly defined as the subjective experience, the ability to actively appreciate the nature of one's self and environment. It corresponds to higher-order neural processes and being aware always requires to be awake first (with some notable exceptions, such as dreaming or ketamine-induced dissociative experiences). Awareness is sometimes subcategorized based on neurophysiological features to provide a functional framework: internal awareness refers to the ability to integrate self-consciousness and process inner thoughts, while external awareness includes the perception of the environment and the integration of stimuli from the outside world (Vanhaudenhuyse et al., 2011). Most physiological and pathological states of consciousness have been described in terms of these components (Fig. 1a).
Theoretical models of consciousness. a Physiological (in blue) and pathological (in red) states of consciousness represented across the two-component scale using arousal and awareness, as presented in the initial model. Adapted from Laureys (2005), with permission. b Pathological DoC and their stages of recovery represented in a three-dimensional model including neuroimaging measures, where motor function, overt cognition and covert cognition are drawn along the axes. Reproduced from Edlow et al. (2020) with permission. REM: Rapid Eye Movement; VS/UWS: Vegetative State / Unresponsive Wakefulness Syndrome; MCS: Minimally Conscious State; (C)LIS: (Complete) Locked-In Syndrome; CRS-R: Coma Recovery Scale – Revised; CAP: Confusion Assessment Protocol; fMRI: functional Magnetic Resonance Imaging; EEG: Electroencephalography.
While this conceptualization has been instrumental to theorize the relationships between states, recent developments seem to indicate that this simplified model may be insufficient to fully encompass the spectrum of consciousness disorders (Bayne, Hohwy, & Owen, 2017). The growing use of techniques probing brain activity and connectivity, such as electroencephalography (EEG), positron emission tomography (PET) or functional magnetic resonance imaging (fMRI), provides new biomarkers that can be indicative of conscious processes. The emerging evidence that a substantial fraction of clinically unresponsive patients is able to demonstrate residual brain functions compatible with (covert) consciousness has added new dimensions of complexity to this framework (Schiff, 2015; Thibaut et al., 2021). It seems therefore appropriate to transition from a unique two-component model to a flexible multidimensional workspace where the variables used to deconstruct consciousness integrate multimodal diagnostic tools. An example of a three-dimensional representation of consciousness is provided by Edlow et al. (2020) (Fig. 1b), allowing the integration of neuroimaging-based signatures of consciousness into the model. Other models using connectedness as a third component have been proposed (Martial, Cassol, Laureys, & Gosseries, 2020) and a single solution fitting all situations appears delusive as a comprehensive model would lose its readability.
Theories of consciousnessIn parallel to these component-based frameworks, theorists in cognitive psychology have proposed mechanical explanations for the neural process generating the conscious experience. This debate has been active for several centuries, with some considering René Descartes to be the first scientist to tackle the “hard problem” of consciousness, proposing a hypothesis that considered matter and mind as separate entities. The “hard problem” was coined to describe the challenge of explaining the nature of subjective experience, as opposed to explaining its neural, cognitive and physiological aspects, which are considered “easy problems” (Chalmers, 1995). Anchored into these historical foundations, the modern “theories of consciousness” have however diverged from the Cartesian dualism to adopt a physicalist approach where all mental events are identical to or direct consequences of physical ones (ontological reduction) (Stoljar, 2021). These theories use different conceptual visions, sometimes overlapping and sometimes mutually exclusive, to meet the empirical observations related to human consciousness. Interestingly, they stem from distinct basic scientific grounds, ranging from computational to quantum physics principles (Block, 2009). While no theory is currently recognized as perfect, some do a better job than others in accounting for the different arguments considered necessary for a theory to be valid, as demonstrated in a recent attempt to provide constraining criteria for theories of consciousness (Doerig, Schurger, & Herzog, 2021). In particular, two main currents have gained the most attention from the neuroscientific community so far: the global neuronal workspace theory and the integrated information theory.
The global neuronal workspace postulates that information is made conscious only when a network of widely-disseminated long-range neurons “broadcast” it across a global workspace (Baars, 2002; Dehaene & Changeux, 2011). Parallel processes compete for this single global channel of access to consciousness, while they are temporarily being stored in a short-term memory buffer (Kouider & Dehaene, 2007).
Building upon phenomenology, the integrated information theory attempts to apprehend the physical properties that a system must possess to display conscious experience, using a computational framework. It theorizes the use of a property called phi, a measure of synergy quantifying information integration by the system, to determine whether a system is conscious or not (Tononi, 2005). Only systems that would possess intrinsic irreducibility, therefore whose conceptual structure could not be reduced to the sum of its parts, (i.e., phi > 0) could claim to be conscious (Tononi & Koch, 2015). Although these measures have not yet been empirically demonstrated, their founders have identified that re-entry mechanisms achieving bilateral communication between deep brain structures and cortical areas were central for the generation of consciousness, notably within a posterior “hot zone” (a parieto-occipital area thought to have crucial implications for the generation of consciousness; Siclari et al., 2017). These feedback loops supported by thalamocortical or cortico-cortical white matter tracts may help achieve the integration of information through sustained attention and short-term memory (Edelman & Gally, 2013). The relevance of the posterior hot zone for consciousness is however not universally accepted as there is an ongoing debate over the location (fronto-parietal or parieto-occipital) of the structures underlying consciousness (Boly et al., 2017). As supporters of distinct theories use a very different conception of consciousness, communication between scientists using common concepts can be problematic in this field, although the global neuronal workspace and the integrated information theories are not mutually exclusive. This hurdle has been a limiting factor for the reproducibility of results, as designing common experiments testing the validity of different theories is challenging. The recent development of structured adversarial collaborative projects on consciousness may change this paradigm, as they aim to directly test opposing hypotheses from different theories and implicate the founding researchers behind them (Melloni, Mudrik, Pitts, & Koch, 2021).
Towards a unified diagnostic classificationAlthough researchers struggle to agree on a common definition of consciousness, a consensual classification of nosological conditions presenting consciousness alterations has been necessary to achieve unequivocal communication on the clinical status of a patient. The most widely used diagnostic categories are currently based on clinical manifestations only (Fig. 2) (American Congress of Rehabilitation Medicine, 1995). This derives partly from historical developments but also from concerns regarding access to paraclinical exams and standardization of their results. Regardless, the names, characteristics and diagnostic criteria for these categories are constantly evolving, as our understanding of consciousness progresses and markers of brain activity become more widely accessible (Zasler et al., 2019). Several researchers and clinicians therefore advocate a profound reform in our current diagnostic system, where patients would be tested across a range of evidence-based behavioral, cognitive and neuroimaging tasks (Bayne et al., 2017).
The behavioral classification of DoC. The most commonly observed etiologies are identified on the left side, with text size corresponding to approximate relative frequency in the DoC population. Illustrative cognitively mediated behaviors are pictured for MCS- (visual fixation), MCS+ (command-following) and EMCS (functional communication). LIS (illustrated with communication based on eye movements) is not a DoC but can often be misdiagnosed as such, and must always be excluded in unresponsive patients. Adapted from Wislowska et al. (2017), under the terms of CC BY Attribution License.
A behavioral diagnosis is obtained following the observation of specific clinical behaviors, in a categorical manner, any single behavior in a category being sufficient to the attribution of the diagnostic label. However, behaviors must be observed in a non-ambiguous way, within the prescribed time window and using the adequate trigger/prompt. No formal requirement has been formulated on the behavioral scale required to assess these behaviors, but both European and American guidelines recommend the repeated use of the Coma Recovery Scale – Revised (CRS-R) for its comprehensiveness to detect the minimally conscious state and its intrinsic test performance (Seel et al., 2010). As the long administration time of the CRS-R can be an obstacle in time-constrained clinical settings, the Short Evaluation of Consciousness Disorders (SECONDs) was recently developed and validated to offer a faster and reliable alternative to assess consciousness when time is limited, by focusing on the most frequently observed signs (Aubinet et al., 2021; Sanz et al., 2021).
Acute care and withdrawalAcute loss of consciousness after brain injury can result either spontaneously from dysfunctions directly related to the neural damage (e.g., in traumatic brain injury) and insufficient blood supply to the brain (e.g., in cardiac arrest), or from sedatives administered to the patient by healthcare staff. Coma is defined as a state of prolonged unarousable unconsciousness with absence of both wakefulness and awareness signs, featuring an absence of reaction to external (auditory, visual, noxious) stimuli (Zeman, 2001). A comatose patient is therefore unable to perform spontaneous movements, display any form of voluntary behavior or vocalizations. A minimum duration of one hour usually distinguishes coma from shorter losses of consciousness such as syncope or seizures (Bassetti, 2014). When coma is not due to sedative agents, it often denotes an impairment of brainstem structures responsible for maintaining arousal, in particular the ascending reticular activating system (Young, 2009).
Whether the loss of consciousness is spontaneous or not, most patients with severe brain injury will receive anesthetics and undergo a transient period of artificial coma to allow the stabilization of hemodynamic function, avoid pain, facilitate mechanical assisted ventilation, carry out necessary surgical operations, and optimize biological functions (Nolan & Abella, 2021). Sedation can also serve neurological specific indications, as it reduces the brain's metabolic rates and its sensitivity to ischemia, possibly limiting the extent of brain injury, it drives intracranial pressure down, which can be life-saving for patients with intracranial hypertension, and it decreases seizure rates (Oddo et al., 2016). On the other hand, the use of minimal sedation and daily sedation interruptions has proven to yield better outcomes in the intensive care for critically ill patients, reducing mortality, length of stay, duration of ventilation, nosocomial infections and costs (Barr et al., 2013; Jackson, Proudfoot, Cann, & Walsh, 2010).
A part of those comatose patients will not survive this acute phase, either due to spontaneous early complications, or from withdrawal of life-sustaining therapy. Early end-of-life decisions are usually taken after the initial acute assessment and the exclusion of confounders, when reliable prognostic markers of poor recovery are present and important brain lesions are deemed incompatible with recovery (Varelas, Abdelhak, & Hacein-Bey, 2008). In particular, the clinical absence of brainstem reflexes (pupillary reflex, corneal reflex, oculocephalic reflex) and the disorganization of sleep-wake cycles or circadian rhythmicity (body temperature, movements) are signs indicative of widespread or brainstem lesions, which should orient the clinician to consider withdrawal of life-sustaining therapies. Confirmatory tests with a high sensitivity to predict poor prognosis can be performed to refine clinical decisions, such as somato-sensory or auditory evoked potentials, blood markers of brain injury, EEG or cerebral oximetry (Callaway, 2018).
Prolonged disorders of consciousness and recoveryMost patients who survive the acute phase will recover signs of wakefulness shortly after withdrawal of sedation, by opening their eyes or demonstrating vegetative nervous system activity. This condition of preserved arousal despite the complete absence of clinical signs of awareness is generally called vegetative state (VS) outside of Europe. This term was coined by Jennett and Plum (Jennett & Plum, 1972) as a reference to the preserved vegetative nervous system, contrasting with the unresponsiveness of the higher cognitive functions located in the central nervous system (Adams & Fins, 2017). Patients in this pathological state can display preserved sleep-wake cycles, normal homeostatic functions and reflexive behaviors, such as eye movements, limb flexion, lip movements, tooth grinding or yawning. Yet, they are unable to exhibit voluntary behaviors or oriented responses to external prompts (The Multi-Society Task Force on PVS, 1994). The “persistent vegetative state” defines patients after 1 month in VS, while the “permanent vegetative state” was used for patients in VS after 3 months in the US and 6 months in the UK for non-traumatic injury, and after 12 months for traumatic injuries (Wade & Johnston, 1999). Given the rare but confirmed reports of late recovery after several years in VS, the latest guidelines recommend the elimination of this term in favor of the “chronic vegetative state” which makes less prognostic assumptions (Giacino et al., 2018). The unresponsive wakefulness syndrome (UWS) uses a more descriptive wording as the VS can be erroneously perceived to refer to a “vegetal” or “vegetable” (Laureys et al., 2010). This taxonomy has been more popular in Europe so far, while northern American guidelines recommend the use of both terms, as the perceived value of each is still an active subject of controversy (Fins, 2019; Kondziella, Cheung, & Dutta, 2019).
The minimally conscious state (MCS) has been formulated to provide a common diagnostic entity for patients who could recover signs of conscious awareness in addition to wakefulness. These behaviors can fluctuate and be strongly limited in amplitude by motor or cognitive constraints, but they must be unequivocal and reproducible (Giacino et al., 2002). The patients however remain unable to functionally communicate by any possible means, and their interaction with the external environment is therefore strongly limited. An initial list of behaviors that warrant the presence of conscious processing and define the MCS (command-following, yes/no responses, intelligible verbalization, and purposeful behavior in contingent relation to relevant stimuli, such as appropriate smiling or crying, reaching for objects, visual pursuit or fixation) has been established (Giacino, Kalmar, & Whyte, 2004), but additional behaviors have been proposed to be added to these diagnostic criteria (Mat et al., 2022), such as habituation to auditory startle (Hermann et al., 2020), sound localization (Carrière et al., 2020), resistance to eye opening (Ommen et al., 2018) or eye blinking rate (Magliacano et al., 2021). As the MCS includes very heterogeneous subgroups of patients, a subcategorization of the MCS has been proposed, where the MCS “minus” (MCS-) denotes patients able to demonstrate low-level language-independent signs of consciousness (visual fixation, visual pursuit, localization to pain, oriented movements) and the MCS “plus” (MCS+) defines patients who show higher-level language-related behaviors (command-following, intelligible verbalization, intentional communication) (Bruno, Vanhaudenhuyse, Thibaut, Moonen, & Laureys, 2011; Thibaut, Bodien, Laureys, & Giacino, 2020).
When patients are able to functionally communicate, either through verbal interaction, a communication code or assistive technology, or when they are able to functionally use common items, they are not considered to suffer from DoC anymore as they have emerged from the MCS (EMCS). This distinction is however subjective as they often remain severely handicapped both physically (e.g., with hemiplegia) and cognitively (e.g., with delirium), and most of them will require intensive neurological rehabilitation and daily assistance for years. As communication resumes, the field of possibilities to test the patients’ cognitive functions widens tremendously. Using adapted versions of neuropsychological tests, an assessment of cognitive deficits can be made to detect frequent symptoms such as aphasia, confusion, memory and attention impairments, dysmetria or spatial neglect (Bodien, Martens, Ostrow, Sheau, & Giacino, 2020; Sherer et al., 2020).
Brain imaging and covert consciousnessUsing standardized assessments such as the CRS-R to diagnose consciousness levels at the bedside is essential to avoid high misdiagnosis rates encountered when using a clinical consensus only (Schnakers et al., 2009). However, using diagnostic categories based solely on clinical findings, even with standardized measures, may still miss a substantial fraction of clinically unresponsive patients who could display signs of consciousness using brain imaging measures (Stender et al., 2014). Our ability to measure the activity of the human brain has exponentially increased in the last years, with major implications for the diagnosis and the prognosis of DoC. For example, measuring brain glucose metabolism by PET can be employed to characterize whole-brain or regional neuronal activity and assess residual function in key areas for consciousness (Stender et al., 2016; Thibaut et al., 2012). Resting-state high-density EEG combined with machine learning classification algorithms have allowed us to identify conscious signatures in the brain's electrical activity beyond human capability, using an affordable and practical method (Chennu et al., 2014, 2017; Sitt et al., 2014). Resting-state fMRI, transcranial magnetic stimulation combined with EEG or functional near-infrared spectroscopy are additional techniques that have further diversified our imaging toolbox and provide reliable measures of brain connectivity, axonal architecture, complexity or hemodynamic response for diagnostic or prognostic purposes (Abdalmalak et al., 2021; Casali et al., 2013; Demertzi et al., 2015).
These neurophysiological and neuroimaging assessment methods have increased our ability to probe “covert” consciousness in patients with DoC, independently of behavioral responsiveness (Sanz et al., 2021b). The use of fMRI allowed to identify a fraction of patients clinically diagnosed as UWS but able to actively respond to mental tasks involving spoken language in a functional neuroimaging paradigm, a condition termed “cognitive-motor dissociation” (Schiff, 2015). A seminal case report first described successful decoding of fMRI brain activation in a single behaviorally unresponsive patient (Owen et al., 2006), and a larger trial screening 54 patients with UWS or MCS identified 5 patients who could willfully modulate their brain activity in the scanner, but only one was able to use a brain-based yes/no communication code (Monti et al., 2010). Patients with covert consciousness recover consciousness faster and show better long-term functional outcomes than classic DoC patients (Jöhr et al., 2020; Pan et al., 2020). EEG studies have also identified brain activation in response to spoken motor commands among clinically unresponsive patients (Claassen et al., 2019; Cruse et al., 2011), as well as a broad preservation of sleep-wake architecture and brain metabolism measured by PET in patients with covert consciousness (Forgacs et al., 2014; Stender et al., 2014). As specific structural lesions or cognitive impairments may preclude patients from providing an interpretable response to the demanding active tasks (Fernández-Espejo, Rossit, & Owen, 2015), the presence of brain responsiveness to external stimuli can also be tested in passive paradigms, where neuroimaging measures are used to probe the changes in brain activity induced by auditory, visual, noxious or tactile stimuli (“covert cortical processing”) (Edlow et al., 2017). Similarly, EEG can be employed to record neural responses to transcranial magnetic stimulation of the cortex, and the complexity of the signal (measured by the Perturbational Complexity Index) has been shown to reliably predict levels of consciousness (Bodart et al., 2017; Casarotto et al., 2016). The application of artificial intelligence based on deep learning to these data further allows to disentangle awareness and arousal levels and better characterize consciousness alterations using the two-component Explainable Consciousness Indicator (Lee et al., 2022). Residual brain activity at rest based on PET whole-brain metabolism constitutes another approach to detect covert consciousness. This method does not require the integration of external sensory signals by the brain, which may be impaired due to neurological lesions despite preserved conscious processing. The generic suffix “*” after a diagnostic category (as in “MCS*”) was introduced to define a diagnosis based on neurophysiological or neuroimaging results (Gosseries, Zasler, & Laureys, 2014; Thibaut et al., 2021). A meta-analysis reported that MCS patients were more likely than UWS patients to demonstrate covert consciousness in active (34% vs. 14%) or passive (55% vs. 26%) paradigms (Kondziella, Friberg, Frokjaer, Fabricius, & Møller, 2016). These figures confirm that a substantial fraction of unresponsive patients could willfully modulate their brain activity and should be carefully assessed with multimodal paradigms before making therapeutic decisions.
Epidemiology and economic impactSevere acquired brain injury causes major impact on global healthcare, with traumatic brain injury ranking first in trauma-related causes of death and disability (Rubiano, Carney, Chesnut, & Puyana, 2015). DoC can arise following acquired brain injury, yet their prevalence is relatively low in the general population compared to other neurological diseases, as a large proportion of patients with brain injury will either recover consciousness or die in the acute phase. The prevalence of coma was recently approximated using a crowdfunding approach, demonstrating higher rates in the USA (31 for 100 000 individuals) than in the UK (7 for 100 000 individuals), despite large confidence intervals precluding any accurate comparison (Kondziella et al., 2022). Two meta-analyses have estimated the prevalence rates of patients with DoC and found UWS to range between 0.2 and 6.1 for 100 000 individuals (n=5 studies), while the MCS was only reported in one study at 1.5 per 100 000 individuals (Pisa, Biasutti, Drigo, & Barbone, 2014; van Erp et al., 2014). These numbers confirm the rare character of patients with DoC, as the highest prevalence estimates remain far beyond the threshold set to be considered as a rare disease, both in Europe (less than 1/2000 people affected1) and in the USA (less than 200 000 people affected in the country, which corresponds to 1/1645 people2). This dichotomy between the public awareness around brain injury in sports or traffic incidents, and the confidentiality of chronic care in DoC, has contributed to the low availability of resources for healthcare professionals in this field and the lack of incentives to develop new treatments to cure coma. Nevertheless, DoC impose a significant financial burden on the healthcare system due to the long-term and daily care required by patients, often for a lifetime.
The prevalence of DoC in the general population is hard to estimate for several different reasons. The lack of national registries combined with the local management of cases without integrated care trajectories preclude the estimation of prevalence rates based on nationwide systematic identification of patients in most countries (a notable exception is the recent national DoC registry in the Netherlands (Driessen, Utens, Ribbers, van Erp, & Heijenbrok-Kal, 2021)). The rare use of unified criteria to define DoC and the variety of diagnostic scales encountered in rehabilitation facilities complicates the task of comparing common data elements across centers. Patients with severe acquired brain injury often follow care trajectories that include several different medical units, wards and institutions, without efficient communication and integrated care planning between them (Hammond et al., 2021). Also, the local policies and decision-making recommendations on life-sustaining therapies and end-of-life decisions have a major impact on the local prevalence rate of patients with DoC, which creates geographical imbalances related to culture, religion, socioeconomic status, rurality and access to paraclinical exams. As an example, the prevalence rate of UWS in the Netherlands has been estimated to be over 30 times lower than in Italy, a contrast that illustrates diverging practices deriving from historical, legal and religious positions on palliative care (van Erp et al., 2014).
To quantify the economic impact of patients with DoC on the healthcare system is a difficult task as measurable medical costs represent only a small part of the total burden, with many indirect or imponderable financial consequences both at the patient and at the caregiver level. A cost analysis on the “permanent VS” in England and Wales has estimated that a typical single patient in long-term care facilities represented in 2011 over 90′000 £ (144′400 $) per year in medical costs only, with higher costs associated with patients at home than those in specialized nursing homes (Formby, Cookson, & Halliday, 2015). In contrast, hospital rehabilitation units, which provide more intensive neurological therapy, usually for shorter periods, generated higher yearly medical costs estimated to 193′000 £ (305′900 $) in 2012 in the UK (Turner-Stokes, Bill, & Dredge, 2012). These figures may likely underestimate the medical costs incurred by typical patients with DoC in other countries, due to the English publicly-funded healthcare system and the higher volume of rehabilitation therapies required by MCS or EMCS patients compared to those with UWS. On top of these costs, the expenditures associated with assistive technologies, adaptations to the patient's environment, transport and loss of profit cause a major financial strain on caregivers, who already undergo important psychological and physical distress (Cruzado & Elvira de la Morena, 2013; Gonzalez-Lara et al., 2021; Moretta et al., 2014; Soeterik, Connolly, Playford, Duport, & Riazi, 2017).
Prognostic considerationsPrognostic estimates on the natural evolution of patients with DoC are major determiners when it comes to decide whether life-sustaining therapies should be maintained. The legal surrogates of patients often request evidence-based data on long-term recovery from medical staff to base their therapeutic decision on. The clinician should therefore discuss with transparency the range of possible outcomes and openly communicate with relatives on the prognostic uncertainty associated with this patient population. Several reasons can be identified for the poor accuracy of our current prognostic estimates in DoC. First, there is only scarce data on the long-term natural evolution of these patients. Conducting successful longitudinal studies is highly challenging due to the common transfer of these patients to their home or to non-specialized nursing homes after the subacute phase, where follow-up is often lost. The lack of appropriate outcome measures is another obstacle to the successful collection of data on prognosis. The Glasgow Outcome Scale – Extended (Levin et al., 2001) is the most commonly used neurological follow-up scale and can be scored via structured phone interviews, but its sensitivity to small changes in the recovery of DoC patients is very low (e.g., a score of 3 can correspond to MCS-, MCS+ or EMCS, which was only partially addressed by a revised version of the scale (Formisano et al., 2019)). The variability between individuals is another key element preventing healthcare professionals to accurately estimate a patient's likelihood to recover. Patients who recover consciousness early after brain injury have better chances to recover satisfactory cognitive abilities, but rare reports of spectacular late improvements among chronic UWS patients complicate the task when prognosticating a clinically unresponsive patient (Dhamapurkar, Rose, Florschutz, & Wilson, 2016; Errante et al., 2019; Estraneo, Moretta, Loreto, Santoro, & Trojano, 2014; Sancisi et al., 2009).
However, a cautious synthesis of relevant prognostic factors can help to orient the appropriate management. Younger age is associated with better outcomes, probably because of lower comorbidity rates and higher brain plasticity (Estraneo et al., 2022), but infants under 2 years old have worse prognosis due to increased vulnerability during brain and skull development. Traumatic injuries tend to produce focal neuronal damage with possible compensation of contralateral or neighboring regions, which leads to better recovery than patients with widespread anoxic or metabolic injuries (Bruno et al., 2012; Katz, Polyak, Coughlan, Nichols, & Roche, 2009). Additionally, it is still unclear whether different etiologies causing DoC produce common dysfunctions in neural networks, or whether they cause very heterogeneous underlying impairments that have seemingly similar effects as they result in a loss of consciousness (Luppi et al., 2021). In addition, prognostic estimates should include the probability of severe or fatal medical complications based on the individual profile of the patient. Risk factors for infections, epilepsy, respiratory or cardiac diseases, thrombosis, hydrocephalus, renal failure, diabetes or other relevant comorbidities should be considered (Arnts et al., 2020; Lejeune et al., 2021; Romaniello, Bertoletti, Matera, Farinelli, & Pedone, 2016).
Recent advances in neurophysiology and neuroimaging have provided reliable prognostic markers based on brain activity, that can be used to complement clinical indicators of outcome. Glucose metabolism assessed by PET (Stender et al., 2014), qualitative or quantitative EEG (Ballanti et al., 2022), and MRI-based diffusion tensor imaging (Velly et al., 2018) have all shown potential to predict long-term recovery of consciousness, and their concomitant use could increase our prognostic accuracy in the future (Golkowski et al., 2017). The implementation of multivariate predictive algorithms based on artificial intelligence and machine learning will likely change the landscape of post-coma prognostication in the coming years, by integrating large amounts of data combining clinical examination and multiple imaging modalities to provide personalized estimates of recovery and management recommendations (e.g., Zheng et al., 2021).
Life-sustaining therapies in DoC should confront the patient's presumed representations with the range of estimated possible functional outcomes, based on evidence-based prognostic factors. Fig. 3 displays the natural evolution of 195 DoC patients up to 2 years after injury, stratified by behavioral diagnosis at admission to rehabilitation (>3 months) and by etiology (Estraneo & Trojano, 2018). It underlines the poor prognosis of patients who have not recovered signs of consciousness in the first months, influenced by etiology (26% of traumatic UWS had improved at 2 years, against 18% for anoxic and 13% for vascular causes). Traumatic MCS patients have relatively good 2-year outcomes, with almost half of cases reaching EMCS, but almost 40% of patients in this category will remain in a state of MCS, with possible small-scale improvements but the inability to communicate. These figures should be discussed with families to reach a consensual management plan free of interpretation biases.
Natural evolution of behavioral diagnosis. The evolution of behavioral diagnosis at different time points up to two years after injury is shown for 195 patients with disorders of consciousness. Patients are stratified by diagnosis at inclusion in neurorehabilitation (>3 months post-injury), showing patients with unresponsive wakefulness syndrome (UWS) on the left and minimally conscious state (MCS) on the right, as well as lesion etiology, showing traumatic brain injury (TBI) in the top row, anoxia in the middle row and vascular lesions in the bottom row. Figure made with data from Estraneo & Trojano, 2018.
Prognosticating accurately the natural course of brain-injured patients is a major challenge, yet the development of safe and reliable treatments that would modify the course of recovery and actively promote consciousness is an even more pivotal goal, that has been an active subject of research for several decades. Despite the tremendous potential impact in terms of healthcare and financial burden, currently available therapies are limited in efficacy and applicability. In particular, the variability of patient profiles and individual responses may be partly responsible for the low success rate of previous clinical trials. It is commonly accepted that intensive neurological rehabilitation focused on the patient's deficits (which may include physiotherapy, occupational therapy, speech therapy and neuropsychology) should be systematically proposed to all patients as early as possible given the safety of these interventions and the observed progress made by patients in therapy (Giacino, Katz, & Whyte, 2013). Timely prevention and management of medical complications such as seizures, hydrocephalus, infections or spasticity is also crucial to offer optimal environmental conditions for recovery (e.g., Arnts et al., 2020). Regarding therapies that aim to directly modulate consciousness and accelerate recovery, they can be divided into five categories based on their mechanism: pharmacological, electromagnetic, mechanical, sensory and regenerative (Edlow et al., 2021). Pharmacological options involving the dopaminergic and GABAergic neurotransmitter pathways have been the most extensively investigated, based on observed deficits in the homeostasis of these neurotransmitters both in animal and human models. Significant improvement in the recovery of patients have been observed in small-sample uncontrolled studies using different agents (e.g., apomorphine, zolpidem), usually with satisfactory safety of use, however these preliminary results need to be further confirmed in larger-sample controlled trials, and the optimal dosage, administration timing and candidate patient profiles need to be better defined. One placebo-controlled, randomized, double-blind trial in 184 patients has revealed significantly higher rates of behavioral recovery among patients treated with amantadine than those treated with a placebo (Giacino et al., 2012), and amantadine was subsequently recommended in the 2018 DoC guideline endorsed by the American Academy of Neurology, but only for TBI patients aged 16 to 65 years old in the time window of 4 to 16 weeks post-injury (Giacino et al., 2018). Electromagnetic therapies involving electric (transcranial direct current stimulation – tDCS) or magnetic (transcranial magnetic stimulation) noninvasive modulation have also demonstrated efficacy to improve responsiveness in a subset of DoC patients, yet with transient and moderate effects in most cases (Barra, Monti, & Thibaut, 2022). Mechanical therapies (focused ultrasound), sensory therapies (auditory, tactile, vestibular) and regenerative therapies (stem cells) have been less actively investigated to date, with promising theoretical advances, proof-of-concept studies or single case reports that will need to be further developed and tested in clinical settings before they can be widely used in routine care. One notable exception among sensory options is music therapy, which has already demonstrated efficacy to improve functional outcomes in a recent meta-analysis, with an excellent safety profile (Li, Li, Hu, & Wang, 2020).
In summary, the future development of efficient, safe and personalized treatments to improve the recovery of consciousness following severe brain injury will require joint efforts by the international community of researchers, clinicians and financial stakeholders, to promote the implementation of large-scale randomized controlled trials. This process will likely involve the testing of combination therapies (e.g., dopaminergic drugs associated with tDCS), the use of patient-centered outcomes, the recognition of covert consciousness, the selective enrollment of individual patient profiles in trials, the development of novel biomarkers indicating therapeutic response, and the creation of a global DoC clinical trials network (Edlow et al., 2021).
The neuroethics of consciousnessPrognostic and therapeutic considerations are not the only elements to consider when making end-of-life decisions for DoC patients. Neuroethics can be relevant to better understand and guide our decision process regarding severely brain-injured patients (Roskies, 2002). The ethics involved in the rapidly-developing field of coma science are a major and often overlooked concern currently resurfacing in the light of functional neuroimaging and other recent neuroscientific developments (Racine, Rodrigue, Bernat, Riopelle, & Shemie, 2010). Medical technologies such as mechanical ventilation, enteral feeding or more recently computer-assisted communication, are artificial substitutes to our vital functions. The question of utility and futility of these medical treatments should remain central and be evaluated on a regular basis in DoC patients. Notably, it is crucial to differentiate therapies that aim to promote consciousness directly (e.g., dopamine agonists) from those that aim to treat associated symptoms (e.g., anti-spasticity drugs). Our limited understanding of brain processes underlying consciousness should warrant extra caution regarding a patient's ability to feel, hear or see external stimuli, even for unresponsive subjects. Whenever possible, all measures to ensure maximal comfort, hygiene and privacy should be taken. The management of pain should be carefully evaluated by paying close attention to signs of discomfort during nursing, especially in MCS patients (Chatelle et al., 2014). The patient should always be addressed directly, carefully explaining all examinations performed. This is particularly important given the large prevalence of patients with covert consciousness (Thibaut et al., 2021), but also to minimize the risk of identity loss and depersonalization commonly reported with this population (Gray, Knickman & Wegner, 2011).
Understanding our cognitive processes and their associated biases is a helpful way to improve our critical judgment when taking ethical decisions (Lejeune et al., 2020). For example, the use of neuroimaging tests only to confirm an initial diagnostic hypothesis (confirmation bias) and the obstinacy to trust this diagnosis despite new contradictory evidence (loss aversion) are commonly encountered in clinical practice, as well as the tendency to overestimate one's prognostic confidence due to diagnostic accuracy (attribute substitution), apparent expert opinion (blind obedience) or apparent consensus (bandwagon effect) (Rohaut & Claassen, 2018).
ConclusionsConsciousness is a multifaceted and versatile concept, and its scientific study has been strongly impeded by the difficulty to achieve a consensual definition for it. As theorists are still actively debating its generating mechanisms in the brain, clinicians faced with patients suffering from severe acquired brain injury must nevertheless adopt practical strategies to characterize altered consciousness and promote recovery. DoC have been classified and treated using essentially behavioral assessments up to today, but a new era of brain activity measures and the evidence on the high rates of covert consciousness are calling for a profound reform of the current taxonomy. The heterogeneity of individual patient profiles calls for the development of precision medicine frameworks using biological biomarkers. Combining the clinician's expertise and evidence-based paraclinical exams, individual prognostication and personalized treatment plans could be offered to patients (Fig. 4).
Multimodal management of brain-injured patients. The combination of reliable clinical assessments and multimodal measures of brain activity allow the realization of an accurate diagnosis of consciousness. The integration of these elements can benefit from advanced computational tools such as artificial intelligence, and should involve neuroethical considerations. Through these processes can be established a modern era of post-coma care based on personalized, patient-centered and evidence-based treatments.
It is therefore fundamental to equip first-line healthcare professionals with a comprehensive toolbox containing practical and interpretable diagnostic indicators, as well as personalized and actionable therapies. We advocate the creation of tight partnerships between research teams and clinical units to keep these tools sharp and translate the neuroscience of consciousness into better post-coma care.
Funding sourcesThis work was supported by the University and University Hospital of Liege, the Belgian National Funds for Scientific Research (FRS-FNRS), the fund Generet, the King Baudouin Foundation, the Fondation Leon Fredericq, the FNRS PDR project (T.0134.21), the FNRS MIS project (F.4521.23), the European Union's Horizon 2020 Framework Programme for Research and Innovation under the Specific Grant Agreement No. 945539 (Human Brain Project SGA3), the European Space Agency (ESA) and the Belgian Federal Science Policy Office (BELSPO) in the framework of the PRODEX Programme, the Center-TBI project (FP7-HEALTH- 602150), the Public Utility Foundation ‘Université Européenne du Travail’, “Fondazione Europea di Ricerca Biomedica”, the AstraZeneca Foundation, the BIAL Foundation, the Mind Science Foundation, the European Commission, the Mind-Care foundation, the Televie Foundation and the DOCMA project [EU-H2020-MSCA–RISE–778234]. LRDS is a research fellow, OG is a research associate, and SL is research director at FRS-FNRS.
CRediT authorship contribution statementLeandro R.D. Sanz: Conceptualization, Methodology, Investigation, Writing – original draft, Visualization, Funding acquisition. Steven Laureys: Conceptualization, Writing – review & editing, Supervision, Project administration, Funding acquisition. Olivia Gosseries: Conceptualization, Methodology, Writing – review & editing, Supervision, Project administration, Funding acquisition.