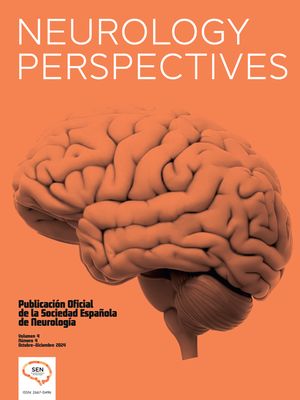
Biomaterial and stem cell -based treatment strategies for diseases of the central nervous system
Más datosParkinson's disease (PD) is the second most frequent degenerative disease and is characterised by dyskinesia, postural instability, and tremor due to selective loss of dopaminergic neurons of the substantia nigra pars compacta (SNpc). Early approaches to the management of PD aimed to alleviate symptoms using pharmacological treatments. However, not all patients tolerate drugs equally and due to the progressive course of the disease, medication needs to be adjusted over time. Furthermore, most patients are elderly individuals with concomitant diseases, which increases the risk of drug–drug interactions. In this context, the discovery of adult neurogenesis has caused a dramatic change in the therapeutic approach, aiming to repair the nervous system. However, cell replacement therapies alone have been unable to solve this situation, as they do not modify the underlying neurodegeneration. For this reason, the solution may lie in combining different approaches. There is a need to identify early biomarkers that may be detectable before onset of neurodegeneration, to identify and apply more appropriate pharmacological treatments for the early stages of the disease, to tailor pharmacological treatments, and to use cell replacement therapies, taking into account the need to reprogram the cells used and to avoid as many adverse effects as possible, including immune reactions and tumours. The future of PD treatment represents a significant challenge due to its heterogeneity.
La enfermedad de Parkinson (EP) constituye la segunda enfermedad degenerativa más frecuente, caracterizada por la discinesia, inestabilidad postural y temblores debido a una pérdida selectiva de neuronas dopaminérgicas de la pars compacta de la substantia nigra (SNpc). El enfoque inicial para de la EP ha consistido en aliviar la sintomatología del paciente mediante el uso de tratamientos farmacológicos. Sin embargo, no todos los pacientes toleran los medicamentos de igual modo y al tratarse de un trastorno progresivo, se hacen necesarios ajustes en la medicación a lo largo de tiempo. Además, la mayoría son pacientes geriátricos con enfermedades concomitantes, lo que puede ocasionar interacciones con otros fármacos. Pero con el descubrimiento de la neurogénesis adulta se genera un cambio drástico en el enfoque terapéutico para la reparación del sistema nervioso. Sin embargo, las terapias de reemplazo celular se han mostrado incapaces de solucionar el problema por sí solas, dado que no modifican la neurodegeneración subyacente. Por este motivo, la solución podría pasar por una combinación de enfoques. Es necesario encontrar biomarcadores tempranos, previos a que se manifieste la neurodegeneración, identificar y aplicar los tratamientos farmacológicos más adecuados para las fases iniciales de la enfermedad, realizar el tratamiento farmacológico de manera personalizada y emplear terapias de reemplazo celular, teniendo en cuenta la necesidad de reprogramar las células empleadas y evitar todos los efectos adversos posibles como reacciones inmunitarias o generación de tumores. El futuro en el tratamiento de la EP constituye todo un desafío dada su heterogeneidad.
As a result of the increase in life expectancy and therefore in the mean age of the population, neurodegenerative diseases have become a major problem worldwide. Significant research efforts are currently being made to study the aetiopathogenesis of these diseases, but as their origins are still unknown, treatment is exclusively symptomatic.
Our understanding of the brain has changed. In the second half of the 20th century, researchers observed the division of glial cells in the mouse brain1; the migration of postnatal neuroblasts from the subventricular zone to the olfactory bulb, showing the neurogenesis process in the adult brain2; and subsequently the presence of neurons born in the dentate gyrus of adult rats3 and the vocal control nucleus of birds,4 as well as the proliferation of progenitor cells. However, endogenous neurogenesis has been observed not only in vertebrates and lower mammals, but also in humans and other primates; this biological process has recently also been described in human adults.5
Although the adult central nervous system presents only a limited capacity for self-repair, as a result of these findings, the brain is no longer understood as a static structure, drastically changing therapeutic approaches in regenerative medicine for the central nervous system. Stimulation of these physiological processes, transforming neural stem cells into specialised neuronal cells, would represent one means of treating neurodegenerative diseases.6 However, the great complexity of neurodegenerative diseases has led to the development of multiple therapeutic alternatives with very varied results.
Parkinson's disease: Pathogenesis and role of protein aggregationParkinson's disease (PD) is the most common neurodegenerative movement disorder and the second most frequent neurodegenerative disease. In Europe, the prevalence and incidence rates of PD are estimated at 108–257 cases per 100,000 population and 11–19 cases per 100,000 person-years, respectively. Risk factors include older age, male sex, and certain environmental factors.7 PD is a progressive neurodegenerative disease characterised by dyskinesia, postural instability, and tremor, caused by selective loss of dopaminergic neurons of the substantia nigra pars compacta (SNpc); it is also characterised by involvement of the nucleus basalis of Meynert8 and the presence of intraneuronal protein deposition, with Lewy bodies and neurites, composed of α-synuclein and a ubiquitin monomer.9 The death of dopaminergic cells of the SNpc disrupts the motor control network of the basal ganglia, causing typical motor symptoms (bradykinesia, tremor, rigidity, and speech and gait alterations).
In healthy tissue, production of α-synuclein is strictly regulated, whereas in PD, accumulation of α-synuclein protein monomers and aggregates is observed.10
Mitochondrial dysfunction, oxidative stress, and microglial impairment are other factors associated with PD and other neurodegenerative diseases.11
Post-mortem studies of the brains of patients with PD have shown a deficiency in the electron transport chain in the substantia nigra.12 The inhibition of mitochondrial respiratory complexes generates neuronal apoptosis mediated by oxidative stress. Mutations in such genes as parkin and PINK1 have been detected in patients with PD.13 As these genes play a vital role in mitophagy, mutations trigger mitochondrial dysfunction and causes autosomal recessive PD.14
Experimental models used in Parkinson's diseaseWith a view to better understanding PD, models have been developed that seek to reflect the disease as reliably as possible; these include yeast, mouse, fruit fly, and non-human primate models. The first approach consisted of direct injection to the central nervous system of 6-hydroxydopamine (6-OHDA) and 1-methyl-4-phenyl-1,2,3,6-tetrahydropyridine to replicate dopaminergic cellular death in the SNpc. This method enabled researchers to determine the effects of blocking dopamine (DA) expression, but did not replicate the underlying neurological pathology. Furthermore, mouse models have shown that overexpression of human risk factors, such as the α-synuclein gene (SNCA), is associated with an age-dependent degeneration of dopaminergic neurons, similar to that observed in the human pathological phenotype.15,16 These findings support the causal role of α-synuclein in disease progression, but currently lack any clinical application.
Treatments for Parkinson's diseaseUntil the recent development of promising strategies involving the use of human embryonic stem cells (hESC) and induced pluripotent stem cells (iPSC) in models of an existing PD state,17,18 advances have been made using treatments with different approaches: pharmacological, surgical, and modification of the course of the disease.
Pharmacological therapiesThe pharmacological approach aims to alleviate PD symptoms, as no traditional drug has shown a neuroprotective effect against the disease19; in other words, they do not act on the underlying neurodegeneration.20
The most effective pharmacological treatment is levodopa, a DA precursor, combined with a peripheral decarboxylase inhibitor.20 Levodopa is the gold-standard treatment, and is highly effective during the first stages of the disease. However, significant issues arise in the long-term, also reducing its therapeutic effectiveness.21 Combination therapy with carbidopa (Lodosyn) and levodopa is usually administered; this prevents or mitigates such adverse effects as nausea. Inhaled levodopa (Inbrija) may be administered to control the symptoms that appear when the effects of oral drugs wear off; likewise, levodopa may be administered directly to the small intestine using a feeding tube (Duodopa).
As the disease progresses, the benefits of levodopa may become less stable and the patient may start to present involuntary movements after taking higher doses of levodopa.
It should be noted that PD usually presents in geriatric patients with concomitant diseases, who are frequently using other drugs, which increases the risk of adverse effects due to possible drug–drug interactions and contraindications.22 Clearly, pharmacological treatment of PD is complex and requires expertise.23 Furthermore, adjustments must be made over time due to the progressive nature of the disease. When symptoms are mild, patients may function adequately without symptomatic treatment; however, the use of well-tolerated agents in early stages and in the absence of functional impairment provides better long-term results.19
The most frequently used drugs are monoamine oxidase-B (MAO-B) inhibitors that interfere with DA degradation, such as selegiline (Zelapar), rasagiline (Azilect), and safinamide (Xadago), which are useful early therapies24; such neuroprotective drugs as MAO-B inhibitors with propargylamine derivatives, which inhibit oxidative deamination of DA, preventing the generation of oxygen free radicals that can damage nigrostriatal neurons and present antiapoptotic properties; and coenzyme Q10, an antioxidant component whose levels are reduced in patients with PD and with proven neuroprotection against neurodegenerative diseases.25 Such catechol-O-methyltransferase inhibitors as entacapone (Comtan), opicapone (Ongentys), and tolcapone (Tasmar) have also been used; these drugs enhance the effects of levodopa, improving its bioavailability, but have no symptomatic effects in the absence of levodopa. Similarly, DA agonists, classified as ergoline derivatives (bromocriptine, pergolide) and non-ergoline derivatives (pramipexole, ropinirole, and rotigotine), have also been used. These drugs are efficacious in monotherapy in mild to moderate cases of PD, and to supplement levodopa.26,27 DA agonists including rotigotine (Neupro) may be administered in patches, or fast-acting apomorphine injections (Apokyn) may be used.
Anticholinergic drugs reduce the effects of the degeneration of nigrostriatal dopaminergic neurons. DA deficiency, which tonically inhibits striatal cholinergic neurons, leads to increased cholinergic activity.28 Several anticholinergic drugs are currently available, including benztropine (Cogentin) and trihexyphenidyl.
Amantadine presents moderate symptomatic effects. Although the precise action mechanism remains unknown, both dopaminergic and non-dopaminergic mechanisms are involved. It is also useful for suppressing levodopa-induced dyskinesia.27 Dyskinesia is treated by reducing doses of dopaminergic drugs or adding amantadine.24
In young patients with prominent tremor, such anticholinergic agents as trihexyphenidyl are useful, although precautions should be taken due to their potential adverse effects, particularly those related to cognition.24 Contrary to the initial therapeutic approach, in which levodopa was avoided in early treatment, recent research does not support this approach.29
DA agonists are associated with a higher overall risk of adverse events.30 The majority of patients with PD simultaneously use several classes of drugs to complement their benefits, limiting high doses and doses related to adverse events.
Surgical treatmentsSurgical treatment, which has contributed to improving our understanding of the pathophysiology of PD, is indicated when pharmacological treatment is insufficient to control symptoms throughout the day. However, its effectiveness is limited to such motor symptoms as bradykinesia, rigidity, tremor, and drug-induced dyskinesia.31
The initial surgical approach to PD was limited to lesion procedures, such as the first pallidotomies32,33 performed to treat motor symptoms. However, deep brain stimulation has since become the predominant therapy, representing one of the most influential advances since levodopa, lowering doses of the drug and improving tremor.34 The procedure is reserved for cases of advanced PD with motor complications and/or drug intolerance that lead to poor quality of life.
Surgical approaches have improved with current imaging techniques (high-field magnetic resonance imaging, tractography, and functional images), optimising surgical precision and reducing adverse effects. However, the current aim is to develop less invasive therapies that do not require open surgery.
Disease-modifying treatmentsThe above-mentioned therapies treat the motor symptoms of PD but do not alter its course; therefore, researchers have aimed to find alternative treatments that may modify the underlying neurodegeneration, such as gene therapy, immunotherapy, or cell replacement therapies.
Gene therapyClinical trials of gene therapy have aimed to alter enzymes and neurotrophic factors in the basal ganglia. It is able to safely induce expression of specific proteins; however, its clinical effectiveness has not met expectations.35 Some animal studies show that gene therapy is viable and safe,36–38 although concerns have been raised due to the introduction of viral material to the brain that may possibly reach surrounding areas. Techniques are being researched to overcome these issues, as well as the use of alternative vectors.39 Infusion of the gene encoding glutamic acid decarboxylase, the enzyme responsible for GABA synthesis, via a virus into the subthalamic nucleus of patients with advanced PD40 caused no adverse events but was associated with improvements, substantially reducing thalamic metabolism restricted to the treated hemisphere. However, this study did not include a control group and the placebo effect can be considerable in PD; therefore, definitive conclusions on the efficacy of the treatment cannot be drawn.
Animal models have shown that the glial cell-derived neurotrophic factor (GDNF) presents neuroprotective, neurorestorative, and neurological rescue effects.41 Whereas intracerebroventricular injection of GDNF42 is ineffective and is associated with significant adverse effects, administration to the putamen using an infusion pump improved the quality of life of some patients.43 However, another trial including a placebo group identified no significant improvements.44
ImmunotherapyImmunotherapy has been postulated as a potential disease-modifying treatment, using specific antibodies targeting misfolded α-synuclein; however, although trials have shown the safety and tolerability of the treatment, its clinical effectiveness seems to be limited.45
Cell replacement therapyThe discovery of adult neurogenesis led to a drastic change in therapeutic approaches to repairing the nervous system; therefore, the next step after neuroprotection is cell replacement therapy.
Clinical trials have been performed in patients with advanced PD showing resistance to pharmacological treatment. Although the optimal candidates for cell transplantation are yet to be identified, young patients with moderate PD may be the most appropriate.46,47
Grafts of foetal mesencephalic tissue are able to decarboxylate and release DA and mediate a delayed restoration of activity in prefrontal areas of motor circuits.48,49 Graft survival and growth has been demonstrated in morphological studies, whereas the presence of synaptic contacts with host striatal neurons has been confirmed in ultrastructural studies.50 Graft neurons may reinnervate the striatum and restore neural transmission through the basal ganglia. However, methodological differences, both in cellular preparation and transplantation, may lead to diverging results. Transplantation of dissociated tissue (cell suspension) seems to be more effective than solid grafts,51 which would cause more inflammation and gliosis. It is also important to consider the distribution, anatomical location, and heterogeneous cellular composition of grafts.
The foetal dopaminergic neurons transplanted into the striatum probably act in two ways52: (i) as pharmacological agents, they release DA and increase concentration of the neurotransmitter in the striatum; and (ii) in a physiological sense, they reinnervate the striatum by axonal outgrowing and synaptic reconnection, with subsequent regulated DA release, which would prevent the onset of adverse effects, including dyskinesias. However, with current cell preparation and transplantation methods, transplanted dopaminergic neurons are likely to act in both ways. Therefore, the main objective of cell therapy may not be limited to correcting DA deficiency; rather, it may also aim to restore or re-establish connectivity and physiology53,54; this is challenging as transplanted cells, in addition to their ectopic location in the brain, present low survival rates. Despite this, the total number of cells exceeds that required for presence of parkinsonism at PD onset.50,55
Several clinical trials at different stages of development are using autologous and non-autologous cells, including hESCs and iPSCs.56 Over the past two decades, DA-releasing cells from the human foetal ventral mesencephalon have been transplanted to patients with advanced PD, obtaining poor results.46,47,57–60
Grafts of foetal ventral mesencephalic cells survive and reinnervate the brain, restoring motor function and causing prolonged symptom improvement. However, poor survival of the graft has been reported in some patients; together with the need for foetal donors, this has led to limited use of this therapy.
Despite the sustained, moderate improvement in Parkinsonian symptoms and the mild reduction in levodopa-induced dyskinesias,57,53 most patients required concomitant treatment with levodopa in order to obtain significant motor improvements; however, due to the presence of dyskinesia secondary to treatment, transplantation is not recommended as a routine therapeutic option.
The inefficacy of foetal grafts in reverting parkinsonism in advanced stages of PD may be explained by progression of the disease, as it spreads beyond the substantia nigra.
The normalisation of neuronal activity in premotor cortical areas suggests a sequential development of events: cell survival, integration, functioning of cells transplanted into the striatum, and long-term motor benefit.48,49 However, this depends on multiple variables such as the surgical technique used, the level of immunosuppression, cellular preparation and survival, and patient selection.
Double-blind, placebo-controlled studies46,47 have shown minimal, transient improvement of parkinsonism in patients with PD undergoing transplantation of foetal dopaminergic cells, in comparison with the sham surgery group; this contrasts with the findings of open trials, in which patients showed sustained improvement for over 10 years. These differences may be explained by the immunosuppressive treatment used. Deficient immunosuppression would impair cell survival due to the presence of inflammatory processes, affecting the viability of transplanted cells and subsequently clinical recovery.
The preparation and composition of the graft are determining factors of effectiveness and survival. Tissue dissection, differences in foetal age, storage after dissection, and the method of dissociation before graft (in pieces or raw cell suspension) may explain the different results obtained. Cell survival may be compromised due to long cold storage times. Transplantation of dopaminergic cells from inappropriate areas, as well as the proportion of serotonergic neurons in the graft, may explain the onset of dyskinesias in animal models.61,62
The integrity of ascending extranigral dopaminergic pathways is essential for achieving a significant and sustained motor benefit. Patients with dopaminergic denervation exclusively affecting the nigrostriatal pathway present sustained improvement of parkinsonism, but if denervation is more extensive, no motor improvement is experienced after transplantation. As a result, grafts may only be useful in the early stages of PD, when the integrity of the ascending dopaminergic pathways is still preserved,63 although this correlation has not been observed in all clinical trials.64
Transplantation of the graft is essential in obtaining the greatest benefits. Restoring dopaminergic levels within the subthalamic nucleus by grafting dopaminergic cells is essential to achieving complete motor recovery, according to some studies.65
However, the use of foetal tissue presents some ethical and practical issues, such as immunological rejection and the difficulties of obtaining tissue, which has led to a search for new sources of dopaminergic neurons for transplantation. Some studies have transplanted carotid body cells, which constitutes a reliable and safe procedure.66,67 Clinical findings showed a mild, non-significant improvement in parkinsonism, but no dyskinesias.
Retinal cells transplanted to the striatum would act as a DA pump, and have been shown to promote cell survival in cultures of dopaminergic cells due to the trophic factors they release. In animal models, retinal cells led to a significant and sustained motor recovery. One study of patients with PD confirmed these data, showing a moderate clinical benefit but no dyskinesias.68,69
Stem cells are another source for cells for transplant. It is possible to induce differentiation into functional neurons with correct cell and regional identity. However, these populations are heterogeneous and require careful purification to prevent uncontrolled proliferation or differentiation into undesired phenotypes. As a result, protocols have been developed in recent years to induce dopaminergic differentiation, but are yet to be well established.
One advantage of stem cell therapies is that they provide trophic signals to help certain cell populations and/or replace lost cells. In addition to the scarce neurogenesis in adult patients, cell survival also represents a serious problem.
Several sources of stem cells have been suggested for PD treatment, with each case presenting pros and cons. The most frequent sources are embryonic stem cells (ESC), mesenchymal stem cells (MSC), iPSCs, and neural stem cells (NSC).70,71,72,73
Mesenchymal stem cellsMSCs are a series of pluripotent stem cells generally isolated from the bone marrow,74 placenta,75 amniotic fluid,76 umbilical cord blood,77 or adipose tissue.78 They present low tumorigenicity and immune response, compared to ESCs and iPSCs, due to the lack of major histocompatibility complex type II molecules.79
Schiess et al.80 underscore the relevance of neuroinflammation in PD pathogenesis and suggest the use of allogeneic bone marrow-derived MSCs as an immunomodulatory therapy.
Neural stem cellsNSCs are multipotent, self-renewing stem cells that may differentiate to such neural cells as dopaminergic cells, oligodendrocytes, and astrocytes.81 NSCs can be collected from foetal, neonatal, or adult brain regions, including the subventricular zone (SVZ), the subgranular zone (SGZ), and the subependymal zone (SEZ) of the lateral ventricles.82–85 Bilateral intraputaminal grafting of dopaminergic neurons derived from the ventral tegmental area leads to clinical benefits in younger patients, but not in elderly patients with PD.46,47
Embryonic stem cellsESCs are pluripotent stem cells derived from the internal cellular mass, and present high proliferative potential to differentiate into cells of the three germinal layers, including dopaminergic neurons.86 They are excellent candidates for generating DA-producing cells with a high proliferative potential, although they do present some issues.
Induced pluripotent stem cellsiPSCs are adult cells that are genetically reprogrammed to create cells similar to ESCs through the expression of genes and cellular factors (OCT4, SOX2, Klf4, c-Myc, NANOG, and LIN28). There are considerable similarities between iPSCs and ESCs, but iPSCs present a practical solution to the problem of the immunogenic response.87 Dopaminergic neurons derived from self iPSC transplants are able to survive in the brain without immunosuppression, and/or improve motor and non-motor symptoms of PD.88–93
Directly induced dopaminergic neuronsTransdifferentiation is defined as the direct transformation of a somatic cell into another cell type without a pluripotent stage.94,95 Fibroblasts can be reprogrammed for direct transformation into functional dopaminergic neurons.96 This technique is faster than obtaining dopaminergic neurons from iPSCs.
In short, several recent clinical trials have shown some advantages and disadvantages; several other trials are currently at different stages of development (Table 1).
Clinical trials using stem cells in patients with Parkinson's disease, extracted from ClinicalTrials.gov.
Full title of the clinical trial | Stage | Location |
---|---|---|
| Completed | Houston, Texas, USA |
| Enrolling by invitation | Shijiazhuang, Hebei, China |
| Recruiting | Amman, Jordan |
| Completed | Mumbai, Maharashtra, India |
| Recruiting | Orange, California, USANew York, New York, USAToronto, Ontario, Canada |
| Recruiting | Minsk, Belarus |
| Recruiting | Houston, Texas, USA |
| Unknown | Suzhou, Jiangsu, China |
| Recruiting | Sugar Land, Texas, USA |
| Unknown | Zhengzhou, Henan, China |
| Not available | Sugar Land, Texas, USA |
| Unknown | Melbourne, Victoria, Australia |
| Withdrawn | Aventura, Florida, USA |
| Recruiting | Westport, Connecticut, USACoral Springs, Florida, USADubai, United Arab Emirates |
| No longer available | Sugar Land, Texas, USA |
| Recruiting | Jakarta Pusat, DKI Jakarta, Indonesia |
| Unknown | Saskatoon, Saskatchewan, Canada |
| Unknown | Guangzhou, Guangdong, China |
| Draft | Bangkok, Thailand |
| Unknown | Mexico City, Mexico |
| Recruiting | Jerusalem, Israel |
Pluripotent and multipotent stem cells present fundamental differences in proliferation and differentiation capacity. Neural stem cells are multipotent and tissue-specific, and may be isolated from the foetal or the adult central nervous system.97 These cells differ from pluripotent stem cells in their limited proliferation capacity and in the regionally restricted number of mature phenotypes that they may generate.98 Neural stem cells proliferate in response to mitogens and can be cultured and propagated in-vitro in aggregates or neurospheres. Progenitor cells in neurospheres maintain the ability to generate neural and glial lineage cells after the removal of mitogens, although after a prolonged in-vitro passage, their differentiation capacity is fairly limited.99
Unlike stem cells from adults, ESCs do not seem to present limitations for differentiation into any type of somatic cell, including mesencephalic dopaminergic cells. Induction protocols and culture systems have been optimised to generate mouse100 and primate dopaminergic cells,101 and hESC lines.102 Other pluripotent stem cells, such as parthenogenetic stem cells,103 stem cells from somatic cell nuclear transfer (SCNT),104 and iPSCs,105,106 may also differentiate into specific somatic phenotypes for application in replacement therapies.
The most recent advances in cell reprogramming and personalised medicine enable physicians to perform cell therapies and specific treatments that were previously out of reach, by using iPSCs that selectively differentiate into dopaminergic neurons. Unlike other models, when using iPSCs, endogenous cellular machinery and transcriptional feedback are preserved; this is essential in such a genetically complex pathology as PD. These techniques are able to generate iPSC lines with specific genetic risk factors, enabling researchers to assess the response to treatment in different genetic subpopulations. iPSC lines may be genetically corrected and transplanted to a patient with a view to restoring function.107 The advantage of iPSCs lies in the fact that they are specific to the patient, reducing the risk of immunological rejection.
Cell transplant strategies for replacing dopaminergic cells are a promising approach, thanks to the use of biocompatible materials. Biomaterials offer a novel approach to stimulating endogenous neurogenesis and may help in the transplantation of neural progenitor cells, providing a tissue-appropriate physical and trophic milieu for the newly integrating cells.108 The use of biomaterials would improve the survival of grafts by providing an appropriate microenvironment for cells, favouring their adhesion and growth. Biomaterials may be used as support for cellular growth and subsequently be transplanted. Numerous biomaterials have been developed, with different characteristics (hydrogels, nanoparticles, self-assembling peptides, nanofibres, and carbon-based nanomaterials).109 Although more studies are needed before they can be used in humans, the results obtained have been very promising, and they therefore represent a potential therapeutic approach for neurodegenerative diseases.
Limitations of cell therapyOne of the most frequent disadvantages of using ESCs and iPSCs is the risk of tumour formation and genomic instability (Table 2). However, MSCs present mild immunogenic responses, due to the lack of major histocompatibility complex type II.79
Advantages and disadvantages of cell therapy (adapted from Ghamgosha et al.130).
Stem cells | Advantages | Disadvantages |
---|---|---|
NSC | 1. Lower tumorigenicity and immune response than ESCs and iPSCs2. Capacity to form neurons, astrocytes, and oligodendrocytes | 1. Risk of graft-induced dyskinesias2. Limited in-vivo differentiation3. Similar symptoms to PD4. Do not differentiate into cells from the three germinal layers |
MSC | 1. Lower tumorigenicity and immune response than ESCs and iPSCs2. Genuine and accessible cell source3. Improve cognitive and motor performance | 1. Limited therapeutic effectiveness in humans2. Do not differentiate into cells from the three germinal layers |
ESC | 1. High proliferative and differentiation potential2. May generate dopaminergic neurons3. May differentiate into cells from the three germinal layers | 1. Risk of tumour development2. Genomic instability3. Immunogenic response and ethical issues |
iPSC | 1. High proliferative and differentiation potential2. May generate dopaminergic neurons3. May differentiate into cells from the three germinal layers4. No immunogenic response or ethical issues | 1. Risk of tumour development2. Genomic instability |
MSCs present relatively poor therapeutic effectiveness in humans. NSCs present low proliferation capacity and differentiation rates, and lose their characteristics after repeated passages.110 The use of ESCs poses several problems. Their genomic instability, immunogenic responses, the possible rejection of the graft, tumorigenicity, and ethical issues represent significant limitations; therefore, clinical trials with ESCs are yet to be performed in patients with PD. While iPSCs present practically no ethical problems, the immunogenic response, graft rejections, and ectopic overexpression of Klf4, Oct4, Sox2, and c-Myc may lead to the formation of breast tumours,111 epithelial cell dysplasia,112 mucinous colorectal cancer,113 and human cancer,114 respectively.
Furthermore, the combination of several viral structures within the host genome may lead to the development of teratoma.115 Induced dopaminergic neurons are unable to express some specific markers of mesencephalic dopaminergic neurons, and the effectiveness of conversion is low (10%).116 Furthermore, fibroblasts in patients with PD may carry genomic mutations.
ConclusionsAll the advances made to date have contributed to our understanding of PD; however, the disease involves dysfunction of multiple systems and neurotransmitters that cause similar symptoms. From a pharmacological perspective, the variability of responses to levodopa may indicate the presence of multiple biochemical mechanisms of neurodegeneration; which would result in a need for diverse treatments with different approaches.117,118
Treatment is currently personalised according to the patient's expectations, level of disability, employment status, and functional and chronological age, the expected effectiveness and tolerability of drugs, and the response to previous PD therapies19; however, many other factors must also be considered. As no early markers of PD have been identified, many dopaminergic neurons of the substantia nigra will already have died by the time motor symptoms manifest. Therefore, it seems logical to consider cell replacement approaches to achieve restoration.
Since the discovery that foetal transplants may revert Parkinsonian signs in mice models,119,120,121 neural transplantation has aimed to replace the neurons lost due to neurodegenerative processes; however, the clinical effectiveness and application of these techniques are limited by the scarcity of donors of human foetal neural tissue.
Both hESCs and iPSCs present unique characteristics that make them the ideal candidates for research into the development of PD.122 Stem cells may adapt to differentiate into a series of cell fates, including SNpc dopaminergic cells that modulate PD at the cellular level.123,124 Induced stem cells present the advantage of being specific to the patient, which could shed light on the individual contribution of each risk factor for the different mutations associated with PD in a phenotypically similar state.
Researchers have applied and widely reviewed the new genetic reprogramming techniques (TALEN, CRIPSR)125,126. The development of gene editing tools enables research into neurodegenerative mutations, and genetic screening will enable us to better understand disease progression and specific treatments. The influence of specific genetic factors may explain part of the variability in patients' responses to pharmacological treatment. These genetic differences would play an important role in drug metabolism pathways, which would explain the different responses to treatment.127,128
Genetic factors, together with sex, have an impact on treatment, as it has been observed that polymorphisms of the dopamine D2 receptor gene have protective effects in men but not in women.129 Genetic differences may also be influenced by epigenetic factors.
The new techniques being developed include optogenetics, magnetogenetics, and sonogenetics, which offer interesting possibilities, such as treatments aiming to provoke genetic alterations and modulation of the brain circuitry.
The growing body of knowledge on PD, together with the development of new techniques, will help in identifying and considering risk factors that trigger the onset and progression of the condition in each patient, enabling us to design a personalised clinical approach that will lead to more effective clinical therapies.
The heterogeneity of PD represents a challenge for therapeutic development, which makes it extremely important to accomplish two essential objectives: to identify early biomarkers that can be detected before neurodegeneration manifests, and to design disease-modifying therapies that may be used in patients diagnosed using these biomarkers, so that the condition may be treated before it is too late.