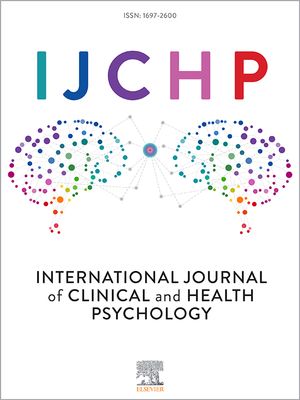
Edited by: Andre R Brunoni, Marie-Anne Vanderhasselt, Leigh Chavert
More infoMajor depressive disorder (MDD) is a serious mood disorder and leading cause of disability. Despite treatment advances, approximately 30% of individuals with MDD do not achieve adequate clinical response. Better understanding the biological mechanism(s) underlying clinical response to specific psychopharmacological interventions may help fine tune treatments in order to further modulate their underlying mechanisms of action. However, little is known regarding the effect of non-pharmacological treatments (NPTs) on candidate molecular biomarker levels in MDD. This review aims to identify molecular biomarkers that may elucidate NPT response for MDD.
MethodsWe performed a systematic review and a multilevel linear mixed-effects meta-analyses, and a meta-regression. Searches were performed in PubMed, Scopus, and PsycINFO in October 2020 and July 2021.
ResultsFrom 1387 retrieved articles, 17 and six studies were included in the systematic review and meta-analyses, respectively. Although there was little consensus associating molecular biomarker levels with symptomology and/or treatment response, brain metabolites accessed via molecular biomarker-focused neuroimaging techniques may provide promising information on whether an individual with MDD would respond positively to NPTs. Furthermore, non-invasive brain stimulation interventions significantly increased the expression of neurotrophic factors (NTFs) compared to sham/placebo, regardless of add-on pharmacological treatment.
ConclusionsNTFs are candidate biomarkers to fine-tune NIBS for MDD treatment.
Major depressive disorder (MDD) is a prevalent mood disorder and one of the leading causes of disability-adjusted life-years worldwide (Diseases & Injuries, 2020). Associated with elevated treatment resistance and recurrence rates, MDD has been linked to poorer quality of life, comorbidity with chronic medical diseases, increased medical costs, as well as a heightened risk of suicide (Dong et al., 2019; Gold et al., 2020; Johnston et al., 2019). As such, MDD is considered a serious public health issue (Diseases & Injuries, 2020).
Current MDD treatment selection relies mainly on symptom examination. First-line standard of care includes pharmacotherapy and/or non-pharmacological treatment (NPT), especially cognitive behavioral therapy (CBT) or interpersonal therapy (IPT) (Guidi & Fava, 2021; Kennedy et al., 2016; Parikh et al., 2016; Park & Zarate, 2019). Second-line treatment involves switching or augmentation of these interventions based on initial patient response (Kennedy et al., 2016; Parikh et al., 2016; Park & Zarate, 2019). Non-invasive brain stimulation (NIBS) treatments such as transcranial direct current stimulation (tDCS) and transcranial magnetic stimulation (TMS) have also been turned to in recent years due to evidence of their ability to modulate cortical activity (Mutz et al., 2018; Nitsche et al., 2009; Zugliani et al., 2021). Pharmacological, psychotherapeutic, and NIBS interventions have been shown to be effective on their own in some capacity to treat MDD (Butler et al., 2006; Cipriani et al., 2018; Cuijpers et al., 2008; Fournier et al., 2010; Mutz et al., 2018; Nitsche et al., 2009; Zugliani et al., 2021), but not without their own limitations (e.g., treatment resistance, side effects, accessibility, and cost barriers) (Kennedy et al., 2016; Milev et al., 2016; Parikh et al., 2016; Park & Zarate, 2019). Despite the number of putative treatment options available, approximately 30% of individuals with MDD do not show significant symptom remission after successive treatment attempts (Rush et al., 2006). To address this barrier, researchers have begun profiling a diverse array of biological markers, or biomarkers, in order to study biological mechanisms underlying the heterogeneity of MDD and variable treatment responses.
Biomarkers are beneficial to clinicians as they provide objective and externally measured information pertaining to diagnosis, disease progress, prognosis, and/or the outcome of a therapeutic intervention (e.g., benefit/harm or lack of benefit/harm) preceding the intervention itself (Gadad et al., 2018). However, there are no validated biomarkers for MDD, which hinders clinicians' ability to offer affected individuals precise and personalized medicine that could optimize symptom remission and increase response rate while simultaneously minimizing the likelihood of harmful side effects. Nevertheless, several molecular biomarkers involved in neurobiological mechanisms theorized to play a role in MDD pathogenesis (e.g., neurotrophic factors (NTFs; e.g., brain derived neurotrophic factor (BDNF)(Bruijniks et al., 2020), glial cell line-derived neurotrophic factor (GDNF)); inflammatory markers (e.g., C-reactive protein (CRP); tumor necrosis factor alpha (TNF-α); and hormones (e.g., cortisol)) have been included in randomized controlled trials (RCTs) in order to assess their influence on MDD symptomatology over the course of treatment (Bruijniks et al., 2020). However, many of these studies are heterogeneous in terms of sample size, treatment type, biomarker detection technique, and participant characteristics. By limiting the scope to non-pharmacological treatments (NPTs), insight may be gained so as how to fine-tune or personalize these interventions for maximal clinical benefit. While recent studies and systematic reviews have investigated molecular biomarkers in predicting MDD treatment response (e.g., Cristea et al., 2019; Gadad et al., 2018; Kennis et al., 2020; Liu et al., 2020), to our knowledge no study has directly compared these biomarkers exclusively across NPT classes. Therefore, the aim of this systematic review and meta-analysis was to provide evidence on candidate molecular biomarkers that may be related to the NPT response in individuals with MDD. We explicitly focus on studies that investigated the effects of NPTs, namely CBT, transcranial electrical stimulation (e.g., tDCS, rhythmic alpha (RAS), rhythmic delta (RDS) stimulation, theta burst stimulation (TBS)), as well as TMS treatments, on the reduction of symptomatology in individuals with MDD.
Materials and methodsSearch strategyThis systematic review and meta-analysis was conducted according to the guidelines outlined by the Preferred Reporting Items for Systematic Reviews and Meta-analyses (PRISMA) statement (Page et al., 2021). The initial article search was conducted in October 2020, and updated in July 2021, within three databases (PubMed, Scopus, and PsycINFO).
The search was performed using the following keywords: “Major depressive disorder”, “MDD”, “major depression”, “depressed”, “depressive”, “biomarkers”, “blood”, “fecal”, “microbio*”, “MRS”, “magnetic resonance spectroscopy”, “immun*”, “hormon*”, “metabolic*”, “neuroendocrin*”, “neurotransmit*”, “protein*”, “neurotroph*”, “gastrointestin*”, “proteom*”, “plasma”, “biomarker*”, “marker*”, “surrogate*”, “serum”, “saliva”, “urine”, “cerebrospinal”, “PET”, “ELISA”, “positron emission”, “cognitive behavioral therapy”, “CBT”, “cognitive behav*”, “transcranial direct current stimulation*”, “transcranial magnetic stimulation*”, “tDCS”, and “TMS”, with “*”accounting for terms with alternative endings. A full description of the search strategy is presented in the Supplementary Material (SM).
Inclusion and exclusion criteriaPeer-reviewed, since first date available, English-language parallel RCTs meeting the following criteria were considered for inclusion: [1] Included adult participants (≥ 18 years of age) with at least group having MDD as a main diagnosis (as defined by recognized diagnostic criteria, such as the Diagnostic and Statistical Manual for Mental Disorders (DSM)); [2] Participants were required to be taking no or stable medication preceding the start of NPT; [3] Conducted repetitive (≥ 5) NPT sessions, such as CBT, transcranial electrical stimulation (e.g., tDCS, RAS, RDS, TBS), or TMS interventions. CBT therapy could be offered in any format (e.g., in-person, online, in groups, etc.), so long as a certified psychologist or psychiatrist was leading the treatments). Control treatments included any other intervention or comparator group arm of the RCT.; [4] Reported molecular biomarker data (i.e., detected via blood, saliva, urine, molecular biomarker-focused neuroimaging methods, etc.) collected both pre- and post-treatment.
The following study exclusion criteria were applied: [1] Participants disclosed to: (a) have a past or present systemic disease/disorder (e.g., diabetes, cardiovascular disease) that was the focus of the study, (b) were pregnant, (c) had suffered a brain injury, (d) were a member of a risk group that was the focus of the study; [2] Participant groups diagnosed with a personality disorder; [3] Participants reported to be dependent on or abusing alcohol and/or illicit drugs; [4] Studies conducting treatment combinations on the same participant(s) (e.g., TMS and then CBT) if the data from a control group (i.e., group that only received one treatment) was not reported; [5] Studies that assessed biomarker data using other methodologies not directly measuring the levels of a specific molecular biomarker (e.g., fMRI, EEG); and [6] Studies that exclusively reported genetic markers (e.g., polymorphism profile).
Quality assessment of included studiesTwo authors (CI and PC) screened the titles and abstracts of articles retrieved from the primary search independently against inclusion and exclusion criteria. The full text of qualifying articles was then assessed against the same standard. Any discrepancies were resolved first through discussion amongst themselves, and if a consensus could still not be reached, by conferring with other group members (SC, JL, BS and ASF). The methodological quality of selected RCTs was conducted independently by two authors (BS and ASF) using the Jadad scale (Jadad et al., 1996). Inter-rater agreement, as determined by Cohen's Kappa coefficient (McHugh, 2012), was verified at each stage prior to resolving disagreements.
Data extractionThe following data was extracted from each study: study groups (i.e., type(s) of intervention and control), percentage of female participants, mean age, description of MDD diagnosis, drug status relating to MDD treatment (i.e., drug-naive, stable medication, tapered medication), treatment duration and frequency, number/duration of sessions, symptomatology assessment and biomarker collection timepoints, main depressive symptomatology assessment (e.g., MADRS), symptom severity change over the trial, and all reported biomarker level data assessed within a treatment arm (e.g., biomarker levels pre- to post- tDCS) and between different treatment arms (e.g., biomarker levels pre- to post-tDCS vs. pre- to post-CBT). Significant effects were defined as having a p-value less than or equal to 0.05.
Mixed-effects meta-analysisTo investigate the magnitude of the effect of NIBS on the change of biomarker levels when compared to sham/placebo conditions and other interventions, the standardized mean difference of the magnitude of biomarker change (i.e., endpoint level minus baseline level) was considered as a dependent variable using the maximum likelihood estimator method, where the standard deviation (SD) of the mean difference was estimated as the average of the SD at baseline and follow-up measurements. Due to the small number of studies (n) that measured the same biomarker, four independent meta-analyses were performed according to the following biological clusters: all interleukins (IL-10; IL-12p40; IL-17α; IL-1β; IL-2; IL-4; IL-5; IL-6), pro-inflammatory interleukins (IL-12p40; IL-17α; IL-6) (Dinarello, 1997), anti-inflammatory interleukins (IL-10; IL-4) (Dinarello, 1997), and NTFs (BDNF; GDNF; NGF; NT-3; NT-4) (Huang & Reichardt, 2001). As BDNF and IL-6 data were reported in multiple studies (n ≥ 3), two additional independent meta-analyses were performed specifically for them. A trial-specific sampling identification (ID) was included as a random effect in all models to account for between-RCT methodological heterogeneity. Additionally, a random effect was included for biomarker type.
Models first investigated the magnitude of NIBS effect size whether or not combined with (+/-) additional treatment when compared to (a) sham conditions +/- placebo treatments, then (b) compared to other interventions (e.g., pharmacotherapy, psychotherapy, etc.). To determine whether the magnitude of the effect of the target intervention group (i.e., NIBS) remained significant independent of additional treatment, heterogeneity was measured by both Cochran's Q and I-squared, and a meta-regression was further performed as an augmentation strategy by considering the additional treatment as a dichotomous variable. In the case of independent intervention groups contributing to multiple different effect sizes (e.g., tDCS +/- psychopharmacological treatment compared to sham tDCS + placebo), the sample size of the shared control group was divided by the number of comparisons (k) with independent intervention groups from the same study to avoid an overweighting of the effect sizes (Higgins et al., 2019). Small study bias and influential cases were investigated by examining the standardized residual for each study and checking for outliers (Biernacki et al., 2016; Viechtbauer & Cheung, 2010). All analyses were performed using the package “metafor” (version 2.0–0) from the open-source statistical software R (version 3.4.3) (Team, 2021; Viechtbauer, 2010).
ResultsStudy characteristicsThe initial search yielded 2146 studies, of which 1260 unique studies were subsequently screened by title and abstract against inclusion and exclusion criteria (Fig. 1). After full-text screening of 62 studies, 17 and six eligible studies were included in the systematic review and meta-analyses, respectively. Upon rerunning a top-up search in July 2021, no new studies were added to either the qualitative or quantitative syntheses. Cohen's Kappa coefficient (McHugh, 2012) was 0.957 (K = 95.7%) and 0.824 (K = 82.4%) for the full-text classification and Jadad methodological assessment, respectively (Table 1).
Study characteristics of included studies.
ID | Authors (year) | Study groups (N) | % Female participants | Mean age (SD) | Drug status | Diagnosis | JADAD |
---|---|---|---|---|---|---|---|
1 | Bruijniks et al. (2020) (48) | CBT 1x weekly (15) | 60 | 38.93 (14.45) | None or stable use (≤3 months) preceding/during trial | MDD or persistent depressive disorder | 3 |
CBT 2x weekly (20) | 65 | 41.70 (10.13) | |||||
IPT 1x weekly (19) | 57.9 | 40.21 (12.63) | |||||
IPT 2x weekly (19) | 63.2 | 41.26 (14.75) | |||||
2 | Li et al. (2018) (26) | CBT (20) | 50 | 27.3 (8.9) | Drug-naïve | MDD | 2 |
SPT (20) | 50 | 26.3 (9.2) | |||||
3 | Moreira et al. (2015) (49) | CBT (28) | 75 | 24.46 (±3.61) | None | Depressive disorder | 4 |
NCT (38) | 81.6 | 23.89 (±3.18) | |||||
4 | Tollefson et al. (1990) (47) | CBT + Imipramine (12) | 76.6 | 33.64 (±10.36) | None influencing HPA besides in intervention groups | Nonpsychotic definite major depressive disorder (unipolar recurrent) | 1 |
CBT (12) | |||||||
Imipramine (11) | |||||||
MST + Imipramine (12) | |||||||
5 | Rahman et al. (2018) (28) | Internet CBT (56) | 76.8 | Median: 49 (IQR: 21) | None or stable use before/during trial | Mild to moderate depression | 2 |
TAU (27) | 77.8 | Median: 48 (IQR: 21) | |||||
6 | Konarski et al. (2009) (46) | CBT (12) | 58.3 | Nonresponders: 26.2 (6.1)Responders: 32.7 (11.4) | None or stable use ≥2–4 weeks preceding trial | MDD | 2 |
Venlafaxine (12) | 58.3 | Nonresponders: 37.8 (12.0)Responders: 40.1 (8.6) | |||||
7 † | Brunoni et al. (2014a)‡ (27) | tDCS + Sertraline (21) | 85 | 41 (13) | None or stable use (≥5 half-life washout) preceding trial, excluding benzodiazepines | Unipolar depressive disorder (without psychotic features, currently experiencing an acute depressive episode) | 5 |
tDCS + Placebo (15) | 66 | 41 (12) | |||||
Sham tDCS + Sertraline (18) | 61 | 41 (1) | |||||
Sham tDCS + Placebo (19) | 63 | 50 (12) | |||||
8 † | Brunoni et al. (2014b)‡ (38) | tDCS + Sertraline (21) | 85 | 41 (13) | None or stable use (≥5 half-life washout) preceding trial, excluding benzodiazepines | Unipolar depressive disorder (without psychotic features, currently experiencing an acute depressive episode) | 5 |
tDCS + Placebo (15) | 66 | 41 (12) | |||||
Sham tDCS + Sertraline (18) | 61 | 41 (1) | |||||
Sham tDCS + Placebo (19) | 63 | 50 (12) | |||||
9 | Brunoni et al. (2015a)‡ (37) | tDCS + Sertraline (21) | 85 | 41 (13) | None or stable use (≥5 half-life washout) preceding trial, excluding benzodiazepines | Unipolar depressive disorder (without psychotic features, currently experiencing an acute depressive episode) | 5 |
tDCS + Placebo (15) | 66 | 41 (12) | |||||
Sham tDCS + Sertraline (18) | 61 | 41 (1) | |||||
Sham tDCS + Placebo (19) | 63 | 50 (12) | |||||
10 † | Brunoni et al. (2015b)‡ (54) | tDCS + Sertraline (21) | 85 | 41 (13) | None or stable use (≥5 half-life washout) preceding trial, excluding benzodiazepines | Unipolar depressive disorder (without psychotic features, currently experiencing an acute depressive episode) | 5 |
tDCS + Placebo (15) | 66 | 41 (12) | |||||
Sham tDCS + Sertraline (18) | 61 | 41 (1) | |||||
Sham tDCS + Placebo (19) | 63 | 50 (12) | |||||
11 † | Brunoni et al. (2018) (25) | tDCS + Placebo (91) | 67.8 | 44.6 (11.9) | Drug-naïve or stable use (≥3–5 weeks) preceding trial, excluding benzodiazepines (≤20 mg/day diazepam equivalent) | Unipolar depressive disorder (without psychotic features, currently experiencing an acute depressive episode) | 5 |
Sham tDCS + Escitalopram (87) | 67.1 | 42.1 (12.5) | |||||
Sham tDCS + Placebo (58) | 67.2 | 41.1 (12.9) | |||||
12 | Pavlova et al. (2018) (50) | 30 min tDCS + Sertraline (27) | 63.0 | 37.0 (8.8) | None or stable use (≥5 weeks) preceding trial, excluding benzodiazepines, antipsychotics, and mood stabilizers | Mild to moderate depression | 3 |
20 min tDCS + Sertraline (21) | 81.0 | 36.0 (10.8) | |||||
Sham tDCS (20) | 75.0 | 40.1 (12.2) | |||||
13 | Zheng et al. (2010) (52) | rTMS (19) | 36.8 | 26.9 (6.2) | Stable escitalopram use (10 mg/day) ≥2 weeks preceding trial and onward | Major depressive episode | 4 |
Sham rTMS (15) | 33.3 | 26.7 (4.3) | |||||
14 | Zheng et al. (2015) (51) | rTMS (18) | 33.3 | 26.9 (6.4) | Stable escitalopram use (10 mg/day) ≥2 weeks preceding trial and onward | Major depressive episode | 5 |
Sham rTMS (14) | 35.7 | 26.9 (4.3) | |||||
15 † | Chou et al. (2020) (53) | TBS (27) | 55.6 | 43.6 (16.6) | None or stable antidepressant, antipsychotic, anticonvulsant use (≥2 weeks) preceding trial; limited use (4 mg/day lorazepam equivalent) of sedatives, hypnotics, anxiolytics | MDD | 5 |
Sham TBS (26) | 65.4 | 42.3 (11.1) | |||||
16 | Zavorotnyy et al. (2020) (44) | iTBS (27) | 48.1 | 40.3 (12.1) | None or stable benzodiazepines and anticonvulsants (washout within 3 half-lives) | Mild unipolar MDD | 4 |
Sham iTBS (28) | 57.1 | 45.6 (12.9) | |||||
17 † | Xiao et al. (2019) (45) | RAS (11) | 45.5 | 32.8 (13.1) | None ≥5 days preceding trial (no fluoxetine during current episode or long-term psychotropic treatment within 6 months); ≥7-day psychotropic medication washout; non-benzodiazepine hypnotics permitted ≤7 consecutive days | MDD | 5 |
RDS (11) | 81.8 | 35.6 (11.3) |
Note. †, studies included in meta-analysis; ‡, studies from same RCT; CBT, cognitive behavior therapy; tDCS, transcranial direct current stimulation; rTMS, repetitive transcranial magnetic stimulation; IPT, interpersonal psychotherapy; SPT, supportive psychotherapy; NCT, narrative cognitive therapy; MST, minimal supportive therapy; TAU, treatment as usual; TBS, theta burst stimulation; iTBS, intermittent theta burst stimulation; RAS, rhythmic alpha stimulation; RDS, rhythmic delta stimulation.
Among the 17 studies included in the systematic review, 906 total participants were nested either among intervention (n = 463) or control (n = 443) study groups (Table 1). The average number of participants included in each RCT was 64.7 (42.0% female participants, 58.0% male participants), with a mean age of 36.1 (range: 24.2–48.5). Intervention groups varied across NPT classes, namely CBT (n = 6), tDCS (n = 6), TMS (n = 2), TBS (n = 2), RAS (n = 1) and RDS (n = 1), alone or in combination with add-on treatment or sham/placebo. Control groups encompassed interpersonal psychotherapy (IPT, n = 1), supportive periodontal therapy (SPT, n = 1), narrative cognitive therapy (NCT, n = 1), exclusive pharmacotherapy (n = 2), pharmacotherapy plus minimal supportive therapy (MST, n = 1), treatment as usual (TAU, n = 1), pharmacotherapy plus sham (n = 6), as well as placebo plus sham (n = 5). To note, the duration, frequency, number of sessions, and treatment protocols were heterogeneous across study groups, even between similar intervention types (Supplementary Table 1).
All studies reported clinical assessments of MDD symptoms, at least at baseline and endpoint. All NPTs, excluding iTBS in Zavorotnyy et al. (2020), were shown to reduce depressive symptoms compared to control interventions (Table 2). Combined CBT plus pharmacotherapy was shown to be the most effective psychological-based NPT in alleviating symptoms. Isolated CBT was also more effective compared to IPT, SPT, NCT, or TAU, however less successful than pharmacotherapy. Among NIBS interventions, tDCS combined with pharmacotherapy was associated with the strongest reduction in MDD severity.
General clinical findings of included studies.
Note. CBT, cognitive behavior therapy; tDCS, transcranial direct current stimulation; rTMS, repetitive transcranial magnetic stimulation; IPT, interpersonal psychotherapy; SPT, supportive psychotherapy; NCT, narrative cognitive therapy; MST, minimal supportive therapy; TAU, treatment as usual; TBS, theta burst stimulation; iTBS, intermittent theta burst stimulation; RAS, rhythmic alpha stimulation; RDS, rhythmic delta stimulation; BDI-II, Beck Depression Inventory-II; HAM-D/HAMD/HDRS, Hamilton Depression Rating Scale; MADRS, Montgomery-Asberg Depression Rating Scale.
Biomarkers of various biological classes were assessed within and between different treatment arms, namely studies investigating neurotrophic factors and/or neurotrophins (n = 6), inflammatory markers (i.e., cytokines, cytokine receptors, C-reactive protein) (n = 6), hormones (n = 2), radiolabeled metabolism markers (n = 1), and other neuronal markers (n = 3) (Table 3). These biomarkers were sourced from various mediums including serum (n = 4), blood (n = 1), urine (n = 1), saliva (n = 1), plasma (n = 6), positive emission tomography (PET; n = 1), and 1H magnetic resonance spectroscopy (1H-MRS; n = 3).
Overall molecular biomarker findings of included studies.
Note. CBT, cognitive behavior therapy; tDCS, transcranial direct current stimulation; rTMS, repetitive transcranial magnetic stimulation; IPT, interpersonal psychotherapy; SPT, supportive psychotherapy; NCT, narrative cognitive therapy; MST, minimal supportive therapy; TAU, treatment as usual; TBS, theta burst stimulation; iTBS, intermittent theta burst stimulation; RAS, rhythmic alpha stimulation; RDS, rhythmic delta stimulation; PET, positive emission tomography; 1H-MRS, magnetic resonance spectroscopy; BDNF, brain-derived neurotrophic factor; CRP, C-reactive protein; TNF-α, tumor necrosis factor alpha; DHEA-S, dehydroepiandrosterone sulfate; IL, interleukin; IFN-γ, interferon gamma; sTNFR(1–2), soluble tumor necrosis factor receptor (1–2); NT-3, neurotrophin-3; NT-4, neurotrophin-4; NGF, nerve growth factor; GDNF, glial cell line-derived neurotrophic factor; m-Ino, myo-inositol; Cho, choline; Cre, creatine; NAA, N-acetylaspartate; Glx, glutamine + glutamate; DOC, dorsal occipital cortex; PHG, parahippocampal gyrus; CER, cerebellum; VACC, ventral anterior cingulate cortex; LPFC, left prefrontal cortex; LACC, left anterior cingulate cortex; ns, not statistically significant (p>0.05); -, not reported; ↑, increased/elevated; ↓, decreased/lowered.
Studies were assessed for associations between changes in biomarker levels pre- to post-NPT with changes in depressive symptoms, and/or correlations between baseline levels of a biomarker and NPT response. Altogether, 13 out of the 17 included studies reported no association between molecular biomarker levels and NPT outcome, and the four studies that reported an association stemmed from groups using molecular biomarker-focused neuroimaging techniques (Konarski et al., 2009; Zavorotnyy et al., 2020; Zheng et al., 2015, 2010). The three main findings reported by the authors were that the increase of Cho/NAA ratio in the anterior cingulate cortex was identified as a significant predictor of attenuation of MDD symptomatology, that the association between changes in Cho/NAA ratio and changes in the attenuation of MDD symptomatology is mediated by the changes in NAA, and that exclusively in the iTBS group, an increased of NAA level during the follow-up period predicted a more noticeable improvement of MDD severity. Altogether, the authors not only suggested that iTBS might increase neuroplasticity, thus facilitating normalization of neuronal circuit function, but suggested that metabolic markers of neuronal viability might be modulated via a possible neurochemical pathway in the anterior cingulate cortex.
No robust associations were described in studies comparing peripheral molecular biomarker levels and NPTs, although Bruijniks et al. (2020) reported that higher baseline serum BDNF levels in individuals with high working memory were related to lower post-treatment depression severity. Furthermore, Tollefson et al. (1990) found that the magnitude of dehydroepiandrosterone sulfate (DHEA-S) change in urine was significantly related to a reduction in depression severity, however this was contingent on pharmacotherapy add-on treatment.
NTFs are candidate molecular biomarkers for NIBS treatmentThe magnitude of the effect of NIBS on changes in molecular biomarker levels was assessed in six studies (n = 6; Table 1) across 69 comparisons (k = 69): all interleukins (n = 3, k = 22), pro-inflammatory interleukins (n = 3, k = 11), anti-inflammatory interleukins (n = 3, k = 6), all NTFs (compared with sham/placebo: n = 3, k = 13; compared with other interventions: n = 4, k = 9), BDNF (n = 3, k = 3), and IL-6 (n = 3, k = 4) (Table 2).
NIBS interventions +/- additional treatment exclusively increased NTF expression compared to sham/placebo (SMD = 0.25; 95% CI: 0.06, 0.44; p = 0.009) (Fig. 2), but not when compared to other intervention arms (e.g., pharmacotherapy, psychotherapy, etc.) (Table 4). This remained the case when considering only tDCS and TBS studies. A meta-regression revealed that this finding remained statistically significant for NIBS interventions independent of add-on pharmacological treatment (NIBS only: SMD=0.28; 95% CI: 0.07, 0.50; p = 0.009; NIBS + pharmacological treatment: SMD=−0.14; 95% CI: −0.59, 0.30; p = 0.527). No significant effects were found for the nested interleukins (i.e., all interleukins, pro-inflammatory interleukins, and anti-inflammatory interleukins) nor for BDNF or IL-6. Egger's regression test for funnel plot asymmetry did not show evidence of publication bias nor between-study heterogeneity for the reported significant finding (Supplementary Fig. 1). Furthermore, no influential cases were identified for the NTF cluster.
Neurotrophic factors mixed-effect meta-analysis (n = 3, k = 13). Note. TDCS, transcranial direct current stimulation; Pharmaco, pharmacotherapy; NT-4, Neurotrophin-4; BDNF, Brain-derived neurotrophic factor; NT-3, Neurotrophin-3; GDNF, Glial cell line-derived neurotrophic factor; NGF, nerve growth factor; GDNF, glial cell line-derived neurotrophic factor.
Meta-analytical findings.
Note. n, number of studies; k, number of comparisons; b, estimate. IL, interleukin; NIBS, non-invasive brain stimulation; BDNF, brain-derived neurotrophic factor; GDNF, glial cell line-derived neurotrophic factor; NGF, nerve growth factor; NT-3, neurotrophin-3; NT-4, neurotrophin-4.
This systematic review and meta-analysis investigated candidate molecular markers that may be related to NPT response in individuals with MDD. Of 2146 screened studies, 17 eligible studies were retrieved for the systematic review, from which six were included in the meta-analysis.
In line with the literature, NPTs were found to effectively reduce depressive symptoms (Butler et al., 2006; Cuijpers et al., 2008; Mutz et al., 2018; Nitsche et al., 2009; Zugliani et al., 2021). Although no consistent correlations were observed between changes in biomarker levels pre- to post-NPT with changes in depressive symptoms, nor associations between baseline levels of a biomarker and NPT response, descriptive findings revealed that brain metabolites accessed via molecular biomarker-focused neuroimaging techniques (i.e., PET, 1H-MRS) may provide promising information on whether an individual with MDD would respond positively to widely-used NPTs. Interestly, it is important to highlight that Li et al. (2018) stated that neuroinflammation in the central nervous system may not be closely related to peripheral immune activation in MDD. In this sense, molecular biomarker-focused neuroimaging techniques may serve as a more sensitive tool to measure molecular biomarker changes modulating mood disorders than biomarkers collected peripherally, for instance, from blood. However, it is important to emphasize that the sample size is low, and the included studies differ in methodology (including the description of “stable” medication allowed over the course of treatment) and means of biomarker collection (Supplementary Table 1). Altogether, it would be beneficial to explore the levels of similar biomarkers derived from molecular biomarker-focused neuroimaging techniques compared to peripheral sources better define the precision and accuracy of each extraction technique. Furthermore, both Bruijniks et al. (2020) and Zheng et al. (2015) highlighted that the examination of biological markers and cognitive profiles may provide a promising approach to understanding the mode of action of NPTs for MDD.
Lastly, the presented meta-analyses suggest that NTF expression is significantly increased following NIBS interventions compared to sham/placebo (SMD = 0.25; 95% CI: 0.06, 0.44; p = 0.009), but not when compared to other intervention arms. This finding is supported by the neurotrophic hypothesis of depression, which postulates that a major depressive episode occurs when neuroplasticity decreases as a result of a deficit in BDNF levels (Karege et al., 2002). BDNF plays a central role in neuroplasticity, as it is related to synaptic strengthening and neuronal survival (Miranda et al., 2019). Its putative role in the NIBS mechanism of action has been explored in several studies, with some reports of elevated blood BDNF levels over the course of a treatment trial (e.g., Yukimasa et al., 2006; Zanardini et al., 2006), while other studies reported no change (Brunoni et al., 2015). These mixed results may be influenced by between-study heterogeneity regarding NIBS treatment type and depression clinical features, such as refractoriness and concomitant use of pharmacotherapy. Although between-study heterogeneity (as measured by Cochran's Q) was not found to be significant within the six studies included in the meta-analysis, it is difficult to draw robust conclusions considering the low sample size mainly stemming from contrasting RCT methodology and varying biomarker collection techniques (Tables 1, 3; Supplementary Table 1). This was the same reason for not performing more biologically relevant separate meta-analyses for all individual biomarkers and wahy additional meta-regressions were not conduced to test the possible confounding effects of sex, age, body mass index, MDD severity at baseline, duration of current depressive episode, and other factors on biomarker levels. Furthermore, the sample size may be biased toward tDCS-specific findings, and a portion of included studies originated from the same overarching RCT (though this was in part accounted for by including RCT-specific sampling IDs in our meta-analysis). Despite significant methodological limitations, this finding may support the association of reduced neuroplasticity with depressive symptomology and therefore may provide insight into the pathogenesis of other conditions involving perturbed neuroplasticity such as post-stroke depression (PSD), in which reduced BDNF levels have been observed (Xu et al., 2018).
In order to identify biomarkers that would provide valuable clinical insight into MDD treatment outcome, there is a need for high-quality studies focused on investigating a wider range of biomarkers and accounting for treatment type. For instance, suitable MDD biomarkers for a corrected thinking or ‘top-down’ approach such as cognitive behavioral therapy (Ruggiero et al., 2018) would likely be different than those for pharmacological treatments relying on molecular mechanisms of action (e.g., serotonin selective reuptake inhibition) (Kennedy et al., 2016), and likewise for NIBS treatments whose exact mode of action are still not fully known (Chan et al., 2021; Noda et al., 2015). Rather than extensively researching isolated and individualized biomarkers hinging on preconceived but incomplete mechanisms, it is critical that future studies work collaboratively and comprehensively in order to search for a more robust link between molecular biomarkers and MDD.
In sum, brain metabolites accessed via molecular biomarker-focused neuroimaging techniques may provide promising information on whether an individual with MDD would respond positively to NPTs. Furthermore, non-invasive brain stimulation interventions significantly increased the expression of neurotrophic factors (NTFs) compared to sham/placebo, regardless of add-on pharmacological treatment. Despite the low number of studies, this data highlights the potential usefulness of molecular biomarkers to better understand the biological mechanism(s) underlying NPTs, which may ultimately drive more fine-tuned treatments and therefore improve therapeutics effects.
This work was partially supported by the Portucalense University. This work was also partially supported by FEDER funds through the Programa Operacional Factores de Competitividade-COMPETE, by national funds through FCT-Fundação para a Ciência e a Tecnologia through the project PTDC/PSI-ESP/29701/2017. It was also supported by grant FCT UIDB/04810/2020 from the Portuguese Foundation for Science and Technology to the William James Center for Research. CLI was supported by Mitacs through a Globalink Research Award (Application Reference IT15520). ASF was supported by FCT and the Portuguese Ministry of Science, Technology and Higher Education, through the national funds, within the scope of the Transitory Disposition of the Decree No. 57/2016, of 29th of August, amended by Law No. 57/2017 of 19 July. The sponsors had no role in the study design, implementation, data analysis or publication.
We would also like to thank Claudia Tirone for her help in conducting some of the background research.
The registered protocol for this systematic review and meta-analysis is available online (INPLASY2021100105; doi: 10.37766/inplasy2021.10.0105).